the Creative Commons Attribution 4.0 License.
the Creative Commons Attribution 4.0 License.
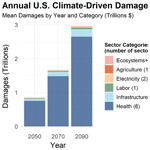
Advancing the estimation of future climate impacts within the United States
Erin E. McDuffie
Karen Noiva
Marcus Sarofim
Bryan Parthum
Jeremy Martinich
Sarah Barr
Jim Neumann
Jacqueline Willwerth
Allen Fawcett
Evidence of the physical and economic impacts of climate change is a critical input to policy development and decision-making. In addition to the magnitude of potential impacts, detailed estimates of where, when, and to whom those damages may occur; the types of impacts that will be most damaging; uncertainties in these damages; and the ability of adaptation to reduce potential risks are all interconnected and important considerations. This study utilizes the reduced-complexity model, the Framework for Evaluating Damages and Impacts (FrEDI), to rapidly project economic and physical impacts of climate change across 10 000 future scenarios for multiple impact sectors, regions, and populations within the contiguous United States (US). Results from FrEDI show that net national damages increase overtime, with mean climate-driven damages estimated to reach USD 2.9 trillion (95 % confidence interval (CI): USD 510 billion to USD 12 trillion) annually by 2090. Detailed FrEDI results show that for the analyzed sectors the majority of annual long-term (e.g., 2090) damages are associated with climate change impacts to human health, including mortality attributable to climate-driven changes in temperature and air pollution (O3 and PM2.5) exposure. Regional results also show that annual long-term climate-driven damages vary geographically. The Southeast (all regions are as defined in Fig. 5) is projected to experience the largest annual damages per capita (mean: USD 9300 per person annually; 95 % CI: USD 1800–USD 37 000 per person annually), whereas the smallest damages per capita are expected in the Southwest (mean: USD 6300 per person annually; 95 % CI: USD 840–USD 27 000 per person annually). Climate change impacts may also broaden existing societal inequalities, with, for example, Black or African Americans being disproportionately affected by additional premature mortality from changes in air quality. Lastly, FrEDI projections are extended through 2300 to estimate the net present climate-driven damages within US borders from marginal changes in greenhouse gas emissions. Combined, this analysis provides the most detailed illustration to date of the distribution of climate change impacts within US borders.
- Article
(3229 KB) - Full-text XML
- BibTeX
- EndNote
Evidence of the physical and economic impacts of climate change is a critical input to policy development and decision-making. Information on the potential magnitude of climate change damages; where, when, and to whom those damages may occur; the types of impacts that will be most damaging; and the potential for adaptation to reduce potential risks are all important and interconnected (Martinich et al., 2018). Understanding this rich set of information can help federal decision makers identify significant climate risks, which is as an important first step toward prioritizing and managing such risks, especially through mitigation and adaptation actions (GAO, 2017). Specifically in the US, results of recent multi-sector impact analyses show complex patterns of projected climate-driven changes across the country, with annual damages in some impact sectors (for example, labor, temperature-related mortality, and coastal property) estimated to range in the hundreds of billions of US dollars by the end of the century (Martinich and Crimmins, 2019; Hsiang et al., 2017).
Climate economics research has also continued to leverage recent advancements to develop and improve our understanding of damage functions that represent climate-driven impacts in broader economic frameworks (NAS, 2017). For example, advances in our understanding of the historical relationships between climatic variables and the economy have enabled the development of methods to assess the economic effects from future climate change within the US (GAO, 2017; Field et al., 2014). As one example, the Climate Change Impacts and Risk Analysis (CIRA) project, coordinated by the U.S. EPA and involving researchers from government, academia, and the private sector, has used and continues to use detailed sectoral models to quantify the physical and economic climate-driven damages across individual impact sectors within the US (e.g., human health, infrastructure, and water resources) (EPA, 2017a). Another example is the Climate Impact Lab – a collaboration of more than 30 climate scientists, economists, and researchers from across the US – which has focused its work on understanding the economic damages from climate change both within the US (Hsiang et al., 2017) and across the globe, including impacts on human health (Carleton et al., 2022), agriculture (Rising and Devineni, 2020; Hultgren et al., 2022), and coastal property (Depsky et al., 2022), and energy (Rode et al., 2021).
Typically, these resource-intensive, bottom-up impact studies rely on a select number of large-scale global emission and warming scenarios (e.g., the Representative Concentration Pathways), limiting their ability to explore certain aspects of uncertainty associated with a wider range of alternative future trajectories. As an alternative approach, the Framework for Evaluating Damages and Impacts (FrEDI) (EPA, 2021b) draws upon information from these detailed sectoral impact studies to rapidly assess US economic and physical impacts of climate change within a common framework. FrEDI was developed using a transparent process and peer-reviewed methodologies and is designed to be a flexible framework that is continually refined to incorporate advances in peer-reviewed economic damage functions, including the incorporation of new sectors and adaptation options. In this analysis, FrEDI draws upon over 30 climate change impact models from peer-reviewed studies to develop relationships between mean surface temperature change and climate-driven impacts across 20 sectors within US borders through the end of the 21st century. FrEDI has the flexibility to use any custom warming scenario (which can be derived from a climate model, e.g., Fig. 1) and couple it with accompanying socioeconomic projections (e.g., gross domestic product (GDP) and population). Due to this level of detail and flexibility, FrEDI provides an efficient and transparent damage estimation approach to explore a variety of future baseline trajectories or emission reduction policies and thereby can provide policy-relevant information and complement the types of analyses and outputs provided by existing integrated assessment models.
In this study, we use 10 000 recently developed paired probabilistic emissions and socioeconomic projections, in combination with resulting temperature projections from a simple climate model, as inputs to FrEDI, which is then run to quantify the annual physical and economic impacts associated with each resulting paired climate and socioeconomic scenario through the end of the 21st century across the contiguous United States (CONUS). This framework allows us to investigate the potential range of projected long-term annual climate change impacts that are associated with uncertainty in climate model parameters, a wide range of future emissions and socioeconomic conditions, and structural uncertainty in select damage functions. We present annual damages over time and discuss the differential impacts projected to occur across different sectors, regions, and populations within CONUS borders to illustrate the breadth of the potential climate change risks to the US. Lastly, we extend our methodology out to the year 2300 to assess the net present damage in the US resulting from an additional tonne of CO2, CH4, or N2O emissions. Aggregating net present damages across all sectors and regions within FrEDI provides a traceable estimate of the economic damages within US borders from a marginal change in greenhouse gas emissions.
This analysis consists of three components, each representing recent scientific advances in their respective fields (Fig. 1). First, projections of global greenhouse gas emissions (Fig. 1, Input 1) are used as input to a simple climate model to derive trajectories of changes in global mean surface temperature (Fig. 1, Output 1). These emission projections were developed as paired scenarios with projections of national-level population and GDP, and the resulting temperature trajectories from the simple climate model are then passed to FrEDI (Fig. 1, Input 2) alongside the paired projections of US population and GDP (Fig. 1, Input 1) to model annual long-term climate damages across 20 impact sectors, seven CONUS regions, multiple adaptation scenarios, and socially vulnerable populations (Fig. 1, Output 2).
Specifically, we use 10 000 randomly sampled scenarios of global greenhouse gas emissions (CO2, CH4, and N2O), US population, and US GDP from the Resources for the Future – Socioeconomic Projections (RFF-SPs) (Rennert et al., 2021) (Sect. 2.1). Emission trajectories are input to the Finite Amplitude Impulse Response (FaIR) model, a simple emissions-based climate model (v1.6.2) that relates emissions to changes in global mean surface temperature (relative to 1850–1900 average) (Smith et al., 2018a). The FaIR calibration is consistent with the IPCC AR6 Working Group 1 assessment of present-day warming, equilibrium climate sensitivity, transient climate response, present-day aerosol radiative forcing, present-day CO2 concentrations, and recent-past ocean heat content change, including the uncertainties in these distributions (Forster et al., 2021; Smith et al., 2021). The resulting 10 000 global mean surface temperature projections, along with corresponding population and GDP projections from the RFF-SPs, are then passed to FrEDI (v3.0) to calculate the physical and economic climate-driven damages. A unique feature of using probabilistic projections with a simple climate model in this approach is the rich range of uncertainty parameters that can be assessed. However, there are some limitations remaining in that separately considering climate parameter and socioeconomic uncertainty ignores potential feedbacks from observed climate change onto socioeconomics (e.g., a higher climate sensitivity could result in larger climate-driven damages, which could lead to lower emissions or GDP than would occur in a lower climate sensitivity world).
We describe each process in more detail below.
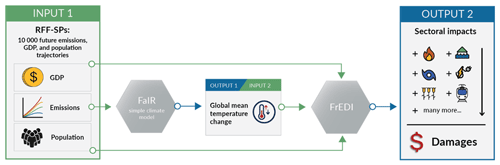
Figure 1Flow diagram of the inputs and outputs needed to evaluate the economic damages within the US emission trajectories are passed as inputs into FaIR to calculate global mean surface temperature. Global mean surface temperature, population, and GDP are then passed as inputs to FrEDI to calculate sectoral climate impacts in the US. Not shown is the estimation of global mean sea level rise; these values are calculated within FrEDI using a semi-empirical approach from existing literature (Kopp et al., 2016) to calculate the impacts to the subset of FrEDI sectors that are impacted by sea level rise (i.e., transportation impacts from high-tide flooding and coastal properties) (EPA, 2021b).
2.1 Emissions and socioeconomics
Socioeconomic and emissions projections from 2020 to 2300 were recently developed under the Resources for the Future Social Cost of Carbon Initiative (Rennert et al., 2021). These include multi-century probabilistic projections of country-level population; GDP; and global emissions of CO2, CH4, and N2O. While uncertainties in multi-century projections are considerable, as discussed in Rennert et al. (2021), these projections represent the largest set of probabilistic socioeconomic and emissions scenarios based on high-quality data, robust statistical techniques, and expert elicitation. These projections also incorporate coupled uncertainty in the time-dependent relationship between GDP and emissions, while also explicitly accounting for potential future climate policy and its contribution to the economy–emissions relationship (Rennert et al., 2021).
2.2 The climate model
The Finite Amplitude Impulse Response model (FaIRv1.6.2)1 calculates atmospheric concentrations of greenhouse gases; radiative forcing; and global mean surface temperature from emissions of greenhouse gases, aerosols, and other gases (Smith et al., 2018a). Version 1.6.2 was calibrated to and extensively used within the Sixth Assessment Report (AR6) of the IPCC (Forster et al., 2021), resulting in 2237 calibrated sets of climate parameters (out of the full 1 million member ensemble). While FaIR only captures uncertainties in those feedbacks and climate tipping points that are apparent in more sophisticated Earth system models or the historic record to which FaIR is calibrated, FaIR does include uncertainties in parameters such as the equilibrium climate sensitivity, transient climate response, present-day aerosol radiative forcing, present-day CO2 concentrations, and recent-past ocean heat content change. Here we use the Monte Carlo simulation capabilities of MimiGIVE.jl (https://github.com/rffscghg/MimiGIVE.jl/releases/tag/v1.0.0, last access: 8 July 2022) to randomly sample the 10 000 RFF-SP emission scenarios (consisting of CO2, CH4, and N2O) and the calibrated set of uncertain parameters contained in FaIR.2 Emissions of the other gases and aerosols (e.g., hydrofluorocarbons (HFCs), black carbon (BC), organic carbon (OC)) not included in the RFF-SP projections were set to the associated emissions in the SSP2-4.5 (Meinshausen et al., 2020) scenario, which most closely matches the median of the RFF-SP emission trajectories (Rennert et al., 2022b). From the 10 000 model simulations, the average change in global mean surface temperature relative to 1986–2005 (FrEDI baseline) is 1.9 ∘C (95 % confidence interval: 0.8 to 3.5 ∘C) by 2100 and increases to 3.1 ∘C (95 % confidence interval (CI): −0.2 to 7.8 ∘C) by 2300 (Fig. A1 in the Appendix).
2.3 Damage from climate change to the US
The Framework for Evaluating Damages and Impacts (FrEDI) is a reduced-complexity model that assesses and quantifies future impacts to the US from a changing climate. As described in detail in the technical documentation (EPA, 2021b), FrEDI uses a temperature-binning approach and data from previously published climate impact studies (Sarofim et al., 2021) to develop relationships between climate-driven changes in CONUS temperature or global mean sea level rise and the resulting physical and economic damages across 20 sectors (Table A1) in seven US regions. While FrEDI evaluates both negative and positive impacts of climate change across sectors and regions, climate-driven damages outweigh the positive effects for all sectors at the national level. FrEDI also provides insight into differences in impacts under various adaptation scenarios and contains a module that can be used to quantify impacts to socially vulnerable populations. The underlying studies in FrEDI consist of bottom-up detailed sectoral analyses from the CIRA project (EPA, 2017a) and other studies including those from the Climate Impact Lab (e.g., Hsiang et al., 2017) and the American Thoracic Society (e.g., Cromar et al., 2022). FrEDI was designed to fill the current need of monetizing a broad range of climate-driven impacts in the US across various warming, emission, and socioeconomic trajectories, while doing so in a significantly shorter computational time frame (e.g., seconds) relative to existing impact models.
FrEDI currently includes 20 impact sectors for which damages are modeled as functions of a climate driver (CONUS temperature or sea level rise), US GDP, and regional population. The GDP and population projections from the RFF-SPs are at the country level (i.e., total US population). For the analysis, we disaggregate national populations from the RFF-SPs to populations for each of the seven FrEDI regions based on the percentage of regional to total US population in the years 2010–2090 using projected regional populations derived from ICLUS (EPA, 2017b). Neither population projections, ICLUS or RFF-SP, were generated considering future climate changes such as climate-induced migration. The proportions for each region are held constant after 2090. Figure A1 shows that the mean and 95th confidence intervals for US population and time-averaged US GDP per capita growth rates are USD 390 million (95 % CI: USD 260 million–USD 520 million) and 1.5 % (95 % CI: −0.4 % to 4.0 %), respectively, in 21003. By 2300, the average of all 10 000 trajectories for US population and time-averaged US GDP per capita growth rates are 370 million (95 % CI: 43 million to 1.3 billion) and 0.9 % (95 %CI: −0.2 % to 3.4 %), respectively. The trends shown in Fig. A1 reflect the aggregate of the 10 000 individual RFF-SP trajectories (each of which has a different but equally likely growth path).
For sectoral impacts driven by temperature change, damages in FrEDI are calculated as functions of CONUS degrees of warming over time relative to a 1986–2005 average temperature baseline. In this analysis, CONUS mean temperature change is estimated for each FaIR-derived temperature projection (calculated from each RFF-SP emissions scenario), as CONUS temperature (∘C) is equal to 1.42 × global temperature (∘C) (EPA, 2021b). This relationship between CONUS and global temperatures is relatively stable across global circulation models (GCMs) and over time, allowing the use of these available data points to develop a generalized relationship between global and CONUS temperature anomalies. Sub-national differences in warming are also explored within FrEDI using results derived from a consistent set of GCMs that were also used within the underlying studies (e.g., Sarofim et al., 2021). For example, unique damage functions for each sector (and variant within each sector) are developed for each region and GCM based on its relationship to CONUS temperature. While FrEDI outputs damages by region and GCM, the main results in this analysis present national and regional damages calculated from the average across the GCM ensemble. For sectoral impacts driven by sea level rise (i.e., coastal properties and transportation impacts from high-tide flooding), global mean sea level is calculated within FrEDI from global mean surface temperature using a semi-empirical method that estimates global sea level change based on a statistical synthesis of a global database of regional sea level reconstructions from Kopp et al. (2016). In FrEDI, sea-level-driven damages are calculated for a given year by interpolating between modeled damages at different sea level heights at that same point in time; this enables FrEDI to account for interactions between adaptation costs, increased coastal property values, and sea level rise over time (EPA, 2021b).
This analysis groups mean damages from each of 20 FrEDI sectors into six topical categories and uses the default FrEDI adaptation assumptions of “reactive”, “reasonably anticipated adaptation”, or “no additional adaptation” (see Table A3) for each sector. As discussed further in Sect. A3, reactive or reasonably anticipated adaptation is where decision makers respond to climate change impacts by repairing damaged infrastructure (e.g., road or rail repair) or reactively responding to current conditions (e.g., building sea walls or beach nourishment) but do not take actions to prevent or mitigate future climate change impacts. No additional adaptation largely incorporates historical or current levels of adaptive mitigation that were in place during the time period of each underlying sectoral study. Example sensitivities to projected climate-driven damages are explored within Sects. 3.1 and A3.
FrEDI also has the capability to investigate adaptation options in select sectors. Available adaptation options reflect the treatment of adaptation in the underlying sectoral studies. For most of these studies, because the implicit or explicit impact response functions are calibrated to historical or current data, historically practiced adaptation or hazard avoidance actions are “baked in”, while enhanced adaptation action or new (currently unknown) technologies are not considered. Exceptions include FrEDI's coastal property and select other infrastructure sectors (e.g., roads, rail), where adaptation options and scenarios from the underlying studies have been incorporated into FrEDI. Total damages in these sectors are sensitive to adaptation assumptions, indicating that adaptation has the capacity to both exacerbate and ameliorate future climate-driven damages, with the latter being more common. These results are further explored below and in Sect. A3.
In addition to quantifying differential climate-driven damages across impact sectors, geographic regions, and adaptation options, FrEDI can also compare climate-driven damages across different populations within the US. This capability is based on a recent EPA Report on Climate Change and Social Vulnerability in the United States (EPA, 2021a), which considers differential climate change risk as a function of exposure to where climate change impacts are projected to occur. These differential impacts are calculated in FrEDI at the census tract level as a function of current population demographic patterns (i.e., percent of each group living in each census tract) (US Census), projections of CONUS population (U.S. EPA, 2017), and projections of where climate-driven damages are projected to occur (from census-tract-level temperature–impact relationships in FrEDI). The relative percent of each group in each census tract is from the 2014–2018 US Census American Community Survey dataset (US Census) and is held constant over time because robust and long-term projections for local changes in demographics out to 2090 and beyond are not readily available. We consider four categories for which there is evidence of differential vulnerability (Table A2), including low income, ethnicity, and race4, educational attainment, and age.
2.4 Estimating net present value of future damages per tonne of greenhouse gas (GHG) emissions
While FrEDI was initially built to project damages through 2090 for temperature scenarios with a maximum value of 10 ∘C of warming, it was extended in this work to project climate damages out to 2300 to quantify the net present damages in the US resulting from an additional tonne of CO2, CH4, or N2O emissions. As described further in Sect. A4, FrEDI is extended by linearly extrapolating its sector-specific, temperature-binned damage functions to account for the full range of temperature scenarios derived from the RFF-SP emission scenarios run through FaIR (some of which have degrees of warming above 10 ∘C). To quantify the net present damages, all 10 000 RFF-SP-derived temperature and socioeconomic scenarios are then run through FrEDI out to 2300 under two cases: a baseline (emissions = RFF-SP emissions) and a perturbed case, where 1 GtC pulse of CO2 (or CH4 or N2O) is added to each of the RFF-SP emissions scenarios in the year 2020. The emissions are identical between the cases for all other years. The annual marginal climate-driven damages are calculated as the difference between the damages in the baseline and perturbed cases, summed across all sectors and all regions for each year. Lastly, these marginal annual damages are discounted to the year of emissions and then aggregated across the time series into a single net present-damage estimate. The results are normalized by the pulse size and gas chemistry (e.g., C to CO2) and reported in 2020 US dollars.
Future monetary impacts are generally discounted relative to present value. Circular A-4 (White House, 2003) recommends a constant value of 3 % for the “social rate of time preference”, which is considered to be the appropriate discount rate to use for impacts on private consumption (which would include most environmental and health impacts). The discount rate of 3 % was calibrated to the real rate of return for 10-year Treasury notes from 1973 through 2003. However, Office of Management and Budget (OMB) Circular A-4 also noted that for intergenerational impacts (a category in which climate change clearly falls), discount rates lower than 3 % might be appropriate. Moreover, recent real rates of return for Treasury notes have been lower than 3 %, adding support for use of a discount rate smaller than 3 % (CEA, 2017). A number of economists, as well as the National Academies of Sciences (NAS, 2017), have alternatively suggested the use of Ramsey discounting (Eq. 2, ρ is the rate of pure time preference, g is a time-varying measure of per capita consumption or income, and η is the elasticity of the marginal value of consumption with changes in gt) as an appropriate approach to discounting long-term problems such as climate change. The effect of Ramsey discounting is to value damages more highly in futures with less economic growth, e.g., future societies that have fewer resources available for adaptation, and vice versa. A recent study from Rennert et al. (2022b) used a Ramsey approach calibrated to a near-term target discount rate of 2 %, with ρ=0.2 % and η=1.24.5 Here we use this Ramsey discounting approach to calculate the net present value.
The net present value (NPV) for a constant discount rate (r) is calculated such that
The net present value for a Ramsey discounting approach is calculated using a time-varying and state-specific discount rate6, which is a function of per capita economic growth (gt):
and where this time-varying rate is then used in the net present value calculation such that
In this expression, gt has also been adjusted to reflect climate damages, such that in any given year gt is the per capita consumption as calculated by taking the exogenous RFF-SP GDP, subtracting the damages output by FrEDI, and dividing by total population. Because most of the sectoral damages as determined from the underlying sectoral models are proportional to GDP per capita (given that the default elasticity of the value of statistical life (VSL) to GDP per capita is 1, all sectors with a mortality endpoint also qualify), a correction can be made to account for this relationship (Nordhaus, 2017). For this analysis, we use the equation
where GDP0(t) is the exogenous RFF-SP GDP, D0(t) is the initial total damages output by FrEDI, and D(t,g(t)) is the resulting damages.
3.1 Annual US climate-driven damages by the end of the 21st century
FrEDI was developed to quantify the physical and economic damages from climate change over the 21st century within contiguous US borders. Figure 2 shows the net annual economic climate-driven damages across 20 sectors in the US in the years 2050, 2070, and 2090, as calculated by the mean from the 10 000 baseline RFF-SP scenarios (i.e., emission, population, and GDP trajectories). Total annual damages throughout this analysis are shown in 2020 US dollars, converted from FrEDI's base units of 2015 USD using annual GDP implicit price deflators (U.S. Bureau of Economic Analysis, 2023). Figure 2 shows that net national damages increase overtime, with mean climate-driven damages estimated to reach USD 2.9 trillion (95 % CI: USD 510 billion to USD 12 trillion), or ∼3 % of US GDP, annually by 2090 for a subset of total climate impacts. Given that the drop in GDP in 2009 during the Great Recession was 2.2 %7, an annual decrease in GDP of over 3.0 % per year by the end of the century (Fig. 3) reflects substantial damage to the national economy (though it is relevant to recognize that much of the damages estimated in FrEDI are a result of mortality, which is not directly reflected in historical GDP estimates). Table 1 provides the 2090 annual mean damages and 95 % confidence interval (CI) for each aggregate category. Confidence intervals presented throughout this section include uncertainty in GDP, population, and climate parameters but do not account for additional sectoral parametric or structural uncertainty. The individual sectors that contribute to each category are listed in Table A1.
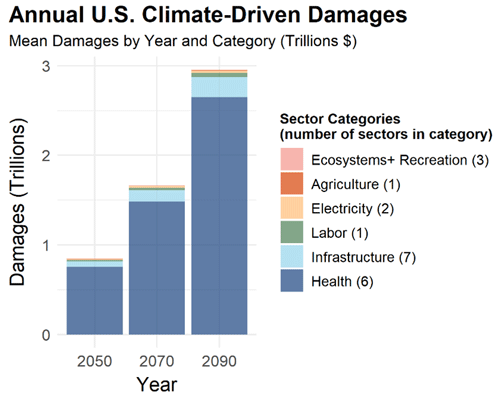
Figure 2Annual mean US climate-driven damages in 2050, 2070, and 2090. Damages are average values in billions of dollars (2020 USD) calculated from the 10 000 RFF-SPs. Sectors are grouped into six categories for visual purposes. The number of sectors included in each category is given in parentheses in the legend. See Table A1 for the list of sectors in each category. Note that this is only a subset of potential climate impacts to the US.
Table 1The 95 % confidence interval (CI) and mean annual US climate-driven damages in 2090 for the six categories shown in Fig. 2. All values are in 2020 USD. Totals may not sum due to rounding.
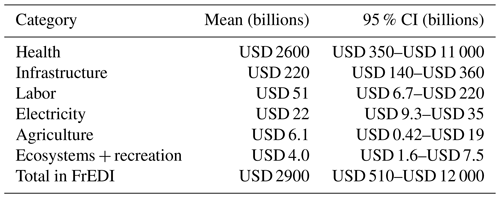
Climate-driven damages from FrEDI are largest for the health category. The majority of damages in this category are from the estimated valuation of premature mortality attributable to climate-driven changes in temperature and air quality (O3 and PM2.5) but also include monetized health damages attributable to Valley fever, Southwest dust, wildfire smoke exposure and suppression costs, and crime incidents. Another FrEDI category that includes the monetized value of directly estimated physical impacts (rather than a direct modeled relationship between temperature and monetized damages) is labor, which is the third-largest category in 2090 and represents the damages resulting from lost hours of work when temperatures are too hot for workers to work outdoors or in unconditioned workplaces (e.g., warehouses). Table 2 provides the mean physical impacts from each of the sectors in the health and labor categories in 2090, along with the 95 % CI. As shown in Table 2, climate-driven changes in temperature have the largest impact on premature mortality, resulting in nearly 50 000 additional deaths (95 % CI: 19 000–91 000 deaths) annually by 2090, followed by climate-driven changes in air quality (5100 deaths; 95 % CI: 2100–10 000 deaths) and exposure to wildfire smoke (1100 deaths; 95 % CI: 460–1700 deaths).
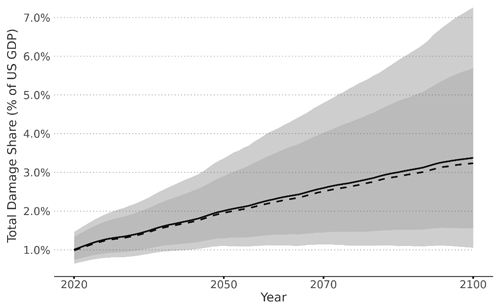
Figure 3Share of US GDP (from the RFF-SPs) of climate-driven damages for those impacts represented in FrEDI. Mean (solid) and median (dashed) lines along with 5th–95th (dark shaded) and 1st–99th (light shaded) percentile bounds.
Table 2The range of 2090 physical impact results across the 10 000 RFF-SP projections, including the 95 % CI and mean. Totals may not sum due to rounding.
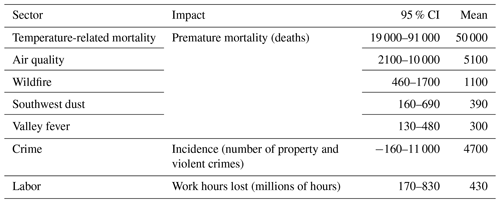
To further illustrate the distribution of monetized damages across sectors, Fig. 4 shows the range of 2090 annual climate-driven damages in each of the 20 sectors in FrEDI, across all 10 000 RFF-SP emission, GDP, and population scenarios, in decreasing order of sectoral mean damages. Figure 4 shows that national total damages in 2090 are primarily driven by the valuation of premature mortality attributable to climate-driven changes in temperature (mean: USD 2.3 trillion per year; 95 % CI: USD 0.31–USD 9.9 trillion per year). The next four sectors with the largest monetary climate-driven damages include premature mortality attributable to changes in air quality (mean: USD 240 billion per year; 95 % CI: USD 32–USD 1000 billion per year), transportation impacts associated with changes in high-tide flooding (mean: USD 140 billion per year; 95 % CI: USD 110–USD 200 billion per year), national labor hours lost (mean: USD 51 billion per year; 95 % CI: USD 6.7–USD 210 billion per year), and health damages from wildfire smoke exposure and response costs from wildfire suppression (mean: USD 51 billion per year; 95 % CI: USD 8.1–USD 220 billion per year). Climate-driven damages to coastal properties associated with changes in tropical storm frequency and wind strength (mean: USD 28 billion per year; 95 % CI: USD 12-USD 49 billion per year), damages attributable to changes in rail (mean: USD 19 billion per year; 95 % CI: USD 7.7–USD 45 billion per year) and road systems (mean: USD 17 billion per year; 95 % CI: USD 6.6–USD 35 billion per year), health damages from changes in southwestern dust exposure (mean: USD 18 billion per year ; 95 % CI: USD 2.5–USD 77 billion per year), and the health burden of change in Valley fever incidence (mean: USD 14 billion per year; 95 % CI: USD 2.0–USD 58 billion per year) round out the top 10 sectors with the largest annual damages in 2090. Figure A2 provides the mean and 95 % confidence interval total damages for each sector over the entire 2020–2100 time series. The large distribution of damages in each individual sector is driven by a large range of RFF-SP emissions, population, and GDP projections and the dependence of the valuation approach for each sector on these parameters (as described in EPA, 2021b).
These sectoral damages are sensitive to assumptions in the adaptation scenarios (see Sect. A3 for more detail). For example, the coastal property sector considers three different adaptation options, no adaptation, reactive adaptation, and proactive adaptation. The underlying model within this sector, the National Coastal Property Model, has options for optimal (“proactive”) response to future sea level rise, “reactive” or reasonably anticipated response to current conditions (including sea walls, beach nourishment, house elevation, or managed retreat), or rebuilding in place as often as necessary. Historical data suggest that most of our response to sea level rise thus far is in between reactive adaptation and no adaptation (Lorie et al., 2020). Considering the range of possible adaptation options in this coastal property sector, mean damages range from USD 17 billion under no adaptation to USD 7.5 billion under proactive adaptation. Damages under the default reactive adaptation assumption are USD 9.4 billion. While the inclusion of adaptation options for any sector within FrEDI depends on the consideration and treatment of adaptation in the underlying impact studies, Table A3 further illustrates that projected climate-driven damages are sensitive to adaptation options in each sector where they are considered. Notably, the largest impact sector in this study, temperature-related mortality, does not include assumptions about future adaptation. While the primary underlying study (Cromar et al., 2022) is a well-regarded meta-analysis of existing global temperature-related mortality studies, it does not explicitly consider future adaptative measures. Exploring projected 2090 damages from one alternative damage function that assesses impacts of extreme temperature on mortality in 49 US cities (Mills et al., 2014) suggests that damages will be reduced (Table A4) in the event that US cities can gradually adapt to hotter temperatures, for example, through physical acclimatization, increased air conditioning penetration, and behavioral changes. Several other studies have also observed reductions in temperature-related vulnerability over time (Lay et al., 2021); however, there is little consensus regarding the most appropriate way to consider future adaptation in this sector, even though several methods have been applied (Sarofim et al., 2016; Carleton et al., 2022; Heutel et al., 2021). Therefore, we use the most recently published meta-analysis for the central estimate in this analysis but also present results from alternative assumptions and studies (Tables A3 and A4), further illustrating the unique advantage of the FrEDI framework of enabling direct comparisons across studies.
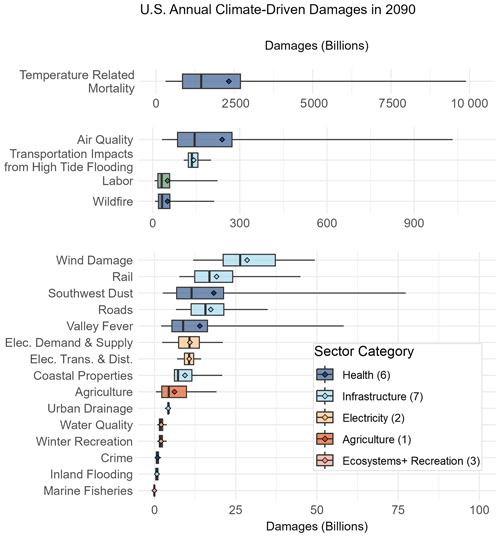
Figure 4Annual US damages in the year 2090 by sector, in order of decreasing mean damages, colored by six sector category groupings. Note the change to the x axis in each panel. Boxes and whiskers show the 2.5th, 25th, 50th, 75th, and 97.5th percentiles and mean damages (diamonds) across all 10 000 projections. Damages are in billions of 2020 USD.
The sectors assessed in this study are independent and therefore damages are additive across these sectors. One potential exception could be temperature-related mortality and the climate–air quality linkage, as most approaches to estimating temperature-related mortality are statistical rather than mechanistic, which could lead to double counting of some health effects between these two sectors. Specifically, Cromar et al. (2022) note that it will be important to continue exploring potential synergies between the effects of temperature and air pollution to adequately capture the potential risk in compound climate events such as these. Conversely, there can also be compounding effects that the FrEDI analytical approach does not account for, e.g., power outages due to increased summer electricity demand could exacerbate temperature-related mortality. However, few studies produce quantitative, monetized estimates of compounding or interacting effects at the national scale as would be required to build into comprehensive impact tools (Clarke et al., 2018).
Results from FrEDI also show that climate-driven damages across the national population vary by geographical region. Figure 5 shows a map of the damages per capita in each CONUS region in the year 2090, with pie charts showing the per capita damages in each region and the share of the four sectors with the largest damages (Fig A3 shows absolute regional damages). Based on the climate impacts included in FrEDI, Fig. 5 shows that the Southeast will experience the largest annual damages per capita (mean: USD 9300 per person annually; 95 % CI: USD 1800–USD 37 000 per person annually), whereas the smallest damages per capita are expected in the Southwest region (mean: USD 6300 per person annually; 95 % CI: USD 840–USD 27 000 per person annually). In each region, the largest monetary damages in 2090 are expected from premature mortality associated with changes in temperature, ranging from USD 4500 per person in the Southwest to USD 6500 per person in the Southeast. Damages from transportation impacts from high-tide flooding and premature mortality attributable to climate-driven change in air quality are the second and third largest in the coastal Southeast and Northeast regions. In the Northwest and Southwest, the sectors with the second- and third-largest climate-driven monetized damages are air quality and wildfires. In the Southern Plains, high-tide flooding transportation impacts and labor hours lost are the second- and third-largest sectors, while rail and wildfires are the second and third largest in the Northern Plains, and labor and rail are the second and third largest in the Midwest. There are some regions and sectors projected to benefit from warming temperatures, including an expected reduction in air pollution attributable mortality in the Midwest under warmer conditions. Overall, however, the negative impacts of climate change outweigh the positives such that net losses are projected in each region.
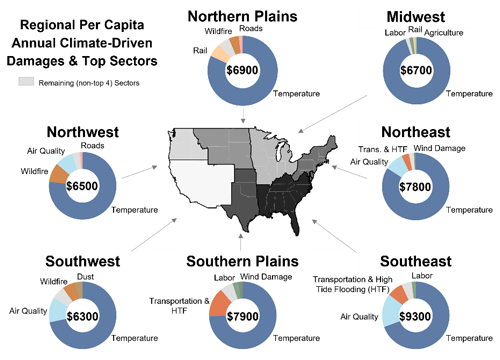
Figure 5Mean per capita annual climate-driven damages across the seven regions in 2090 for the subset of climate impacts included in FrEDI. Donut charts show the annual per capita damages (2020 USD per person annually) and the top four sectors with the largest damages in each region. All damages from the remaining (non-top-four) sectors are shown by the light gray wedges.
Lastly, climate change may also broaden existing societal inequalities (EPA, 2021a), and understanding the comparative risks to different populations is critical for developing effective and equitable strategies for responding to climate change. As described in Sect. 2, FrEDI contains a module to generate and report results of disproportionate exposure and distributional physical effects across four groups of potentially socially vulnerable populations for six sectors. For example, results from this module show that Black or African Americans are more likely to be affected by additional premature mortality from climate-driven changes in air quality, while Hispanic or Latino Americans are more likely to experience lost labor hours (Fig. 6) under a changing climate.
Confidence intervals presented throughout this analysis account for uncertainty associated with the range of future emission and socioeconomic projections across the 10 000 RFF-SP scenarios. These also incorporate climate parameter uncertainty as a Monte Carlo approach was used to sample the calibrated parameter set when running FaIR with the 10 000 RFF-SP emissions scenarios. In addition to these uncertainties and sensitivities to adaptation options, damage estimates within FrEDI are also sensitive to uncertainties in the underlying damage functions themselves. Similar to adaptation, FrEDI can incorporate parametric uncertainty in each damage function when the relevant information is available in the underlying study, as well as structural uncertainty when multiple damages functions are available for a single sector. For example, as further described in Sect. A4, FrEDI incorporates three studies of climate-driven temperature-related mortality, two of which include underlying uncertainty estimates. As shown in Table A4, there is a large range of damage estimates from temperature-related mortality across each study; however, these values all fall within the uncertainty range derived from the RFF-SP scenarios presented in the main text.
3.2 Comparison with Shared Socioeconomic Pathways
To place mean damages in the context of alternative future storylines, Table 3 shows a comparison of annual national climate-driven damages in the US in the year 2090 from a subset of four Shared Socioeconomic Pathways (SSPs), which represent projected socioeconomic global changes up to 2100 (Riahi et al., 2017). Annual damages in Table 3 are calculated following the same approach as outlined in Fig. 1 but using SSP trajectories of emissions, US GDP, and US population from the SSP Public Database (v2.0)8. These trajectories do not include uncertainty related to climate, and thus we only present one value for each trajectory. Table 3 shows that annual US climate-driven damages in 2090 from all but the SSP5-8.5 scenario fall below mean US annual damages as predicted by the RFF-SP scenarios (USD 3.1 trillion). However, annual damages from all SSP scenarios fall within the 95 % confidence interval (USD 0.5–USD 12.3 trillion).
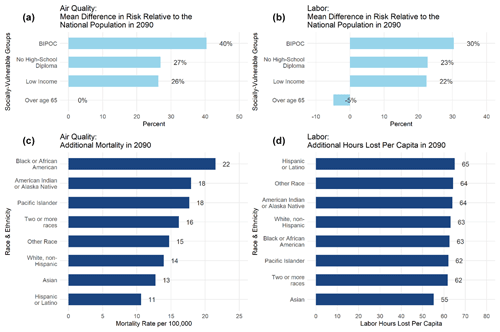
Figure 6Vulnerability to climate-driven changes in air-quality-attributable mortality and labor hours lost, by race and vulnerable groups in 2090. (a, b) Difference in risk in 2090 for four vulnerable populations. (c, d) Additional rates of impacts in 2090, by race and ethnicity.
3.3 Net present damages per tonne of GHG emissions
We extend FrEDI to project climate damages through to 2300 (Sect. A4, Table A5) to quantify the net present damages within the US resulting from an additional tonne of CO2, CH4, or N2O emissions.9 As described in Sect. 2.4, the net present value is the discounted sum of a stream of future damages produced by an emissions pulse in 2020 over the entire 2020–2300 time period. We explore the sensitivity of the remaining estimates to discounting assumptions by using Ramsey discounting calibrated to near-term target rates of 1.5 %, 2.0 %, and 2.5 %. Figure 7 shows the average, median, and range of estimated values for each discounting approach.10
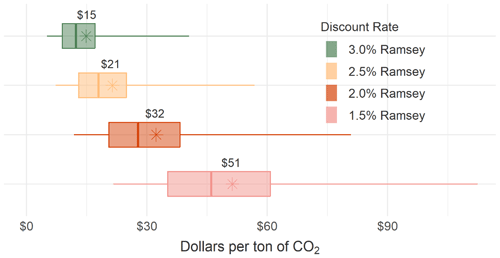
Figure 7Net present value of future damages from 1 t of CO2 for damages occurring only within the CONUS. Units are in dollars (2020 USD) per tonne of CO2 emitted. Whiskers represent the 2.5th and 97.5th percentiles, while boxes span the 25th to 75th percentiles. Mean values (stars and text) and median values (vertical lines) are also shown.
These results show that even considering only the direct CONUS impacts as estimated by FrEDI, damages per tonne of CO2 are almost 20 % of a recently estimated global value (USD 185 per tonne of CO2 under a 2 % Ramsey discounting, Rennert et al., 2022b). This methodology can also be extended to explore the net present value of future damages resulting from an additional tonne of CH4 (USD 500 per tonne of CH4 under a 2 % Ramsey discounting), N2O (USD 9700 per tonne of N2O under a 2 % Ramsey discounting), or other greenhouse gas emissions.
We recognize that multi-century projections are inherently challenging. This is particularly true for socioeconomic projections of GDP, population, and technologies: even projections to the end of the century have been challenged (Barron, 2018). The climate system is better understood, but FaIR only captures the effects of those feedbacks and tipping points that are apparent in the GCMs and historic record to which FaIR was calibrated.
While the damages estimated within FrEDI are constrained to the 48 contiguous United States, it is important to note that the appropriate climate damages to consider when evaluating policy-induced changes in a global pollutant such as greenhouse gases would be damages that account for impacts around the globe. For example, The National Academies of Sciences advised that “[i]t is important to consider what constitutes a domestic impact in the case of a global pollutant that could have international implications that affect the United States” (NAS, 2017). Impacts that occur outside of US borders (and outside of FrEDI) will impact the welfare of US residents and firms because of the interconnectedness of the global economy, international markets, trade, tourism, national security, political destabilization, additional spillover effects, and many other activities not yet captured in FrEDI. Moreover, the act of international reciprocity has been highlighted as motivation for including damages occurring outside of US borders in a social cost estimate of global pollutants (Carleton and Greenstone, 2022; Revesz et al., 2017; and references within). It has also been shown that accounting for global damages in domestic policymaking can be individually rational (Kotchen, 2018). Therefore, we emphasize the contribution of the damages estimated within FrEDI as providing a useful understanding of the channels through which climate change can affect US citizens and residents and their relative magnitudes beyond what is currently possible in many global models yet remain a partial estimate of the total damages from greenhouse gas emissions.
This study presents an evolving framework to quantify the damages of climate change to the US economy, relying on more than a decade of research exploring individual sectoral impacts within the contiguous US (EPA, 2021b). Impacts are dependent upon a change in global mean surface temperature, US GDP, and US population and assumptions about adaptation. Adaptation is relevant in many sectors when quantifying benefits (Sect. A3); however, there are some sectors within FrEDI that do not have explicit options to model adaptation. For example, the largest sector, premature mortality from temperature changes, dominates the monetized damages across all regions. The mortality approach used in this paper is based on a well-regarded systematic review and meta-analysis of temperature-related mortality studies (Cromar et al., 2022). However, there is substantial uncertainty based both on the difficulty of relating historical mortality to temperature changes and the potential for future adaptive responses to reduce vulnerability to temperatures (Carleton et al., 2022; Lay et al., 2021).
While this work advances our understanding of climate-related impacts in the US, it is far from a comprehensive accounting of sectoral damages within the US. The FrEDI framework is dynamic, with new sectors being added to the framework on a continuous basis (including in the near-term several types of health impacts including mental health, vibriosis, and health impacts of extreme storms), as well as broader coverage of direct and indirect impacts of inland flooding. However, the framework still omits coverage of many non-market sectors such as biodiversity, ocean acidification, many other ecosystem service losses, climate-forced migration, conflict. We anticipate that the inclusion of more sectors will increase the estimates of net present damages due to GHG emissions. This work also omits the impacts of tipping elements due to climate change, which may lead to abrupt and irreversible impacts (Armstrong McKay et al., 2022). This study does not explore tipping elements like permafrost thaw or Antarctic ice sheet instability. Future work may entail coupling BRICK to the framework to better explore the uncertainty within sea level rise (Wong et al., 2022, 2017) or coupling to an alternative reduced-form climate model, Hector, to explore permafrost thaw (Woodard et al., 2021). Without explicit representation of some of these feedbacks, we can view these results as potentially lower-bound damage estimates. While CO2 fertilization effects are included in the damage estimates for the agriculture sector, the work does not account for any other direct effects of GHGs, such as the health, agriculture, or ecosystem damages resulting from ozone produced by methane's reaction in the atmosphere. Lastly, this work does not account for interactions among sectors, interactions between non-US and US damages through global markets, and their feedback on the US economy. While we focus on US damages, we acknowledge that impacts resulting from GHG emissions, regardless of where they originate, are global in nature. The bulk of the economic damages from climate change will be outside of the US and the US may also experience indirect effects through trade, business, migration, etc. (NAS, 2017; Hsiang et al., 2017).
Regardless of these limitations, this work significantly advances our understanding of the impacts from climate change to the US, in what US regions impacts are happening, what sectors are being impacted, and which population groups being impacted the most. These results imply that there can be significant benefits to the US from greenhouse gas mitigation, and significant benefits to the people of the US FrEDI can also quantify the benefits of mitigation policies by comparing two scenarios similar to the results presented in Sect. 3.3. Due to FrEDI's flexible framework, it allows for the model to be continually updated as studies of impacts on new sectors or updates to outdated sectoral studies become available. Since this work incorporates multiple disciplines, emission projections, climate modeling, impact modeling, and economic communities, it has the potential to be a useful tool in bridging the research gap between these communities and helping to address some of the omitted climate change risks currently within this field (Rising et al., 2022).
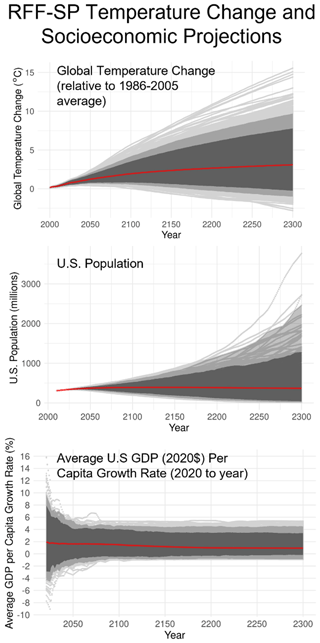
Figure A1Time series of global mean temperature (∘C) relative to 1986–2005 baseline, US population (millions), and average US GDP per capita growth rate (2020 USD) for the 10 000 RFF-SP scenarios from 2020 to 2300. Temperature trajectories are derived from FaIR model runs of the 10 000 RFF-SP emission scenarios. Individual scenarios are shown by light gray lines. Medium and dark gray-shaded regions represent the 99th and 95th percent confidence intervals, respectively. The red line is the mean value over time.
Table A1National annual damage statistics (mean and 95 % confidence interval) for the year 2090, in billions of 2020 USD, listed alphabetically by sector.
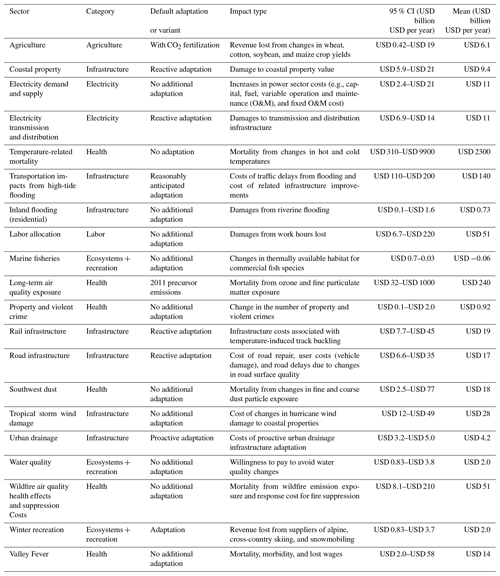
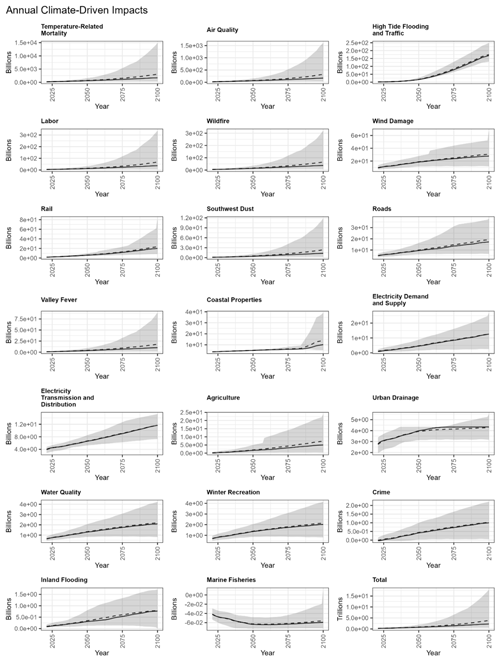
Figure A2Time series of sectoral damages in billions of 2020 USD across all 10 000 projections through 2100 ordered by decreasing mean damages in the year 2090. Total damages (trillions) from all sectors are given in the lower-right panel. Lines show annual mean (dashed) and median (solid) damages. Shaded areas show the 95 % CI. Temporal trends are a function of the underlying temperature (or sea level rise) binned damage functions and sector-specific scalars (e.g., per capita income-dependent VSL). Slight discontinuities in some of these sectors (e.g., agriculture) can occur either at the boundary between temperature bins (e.g., for agriculture and wind damage) or due to thresholds in the underlying studies. For example, the sharp increase in damages in the coastal property damage sector after 2080 correspond to a sharp increase in damages that occur after sea levels breach 100 cm.
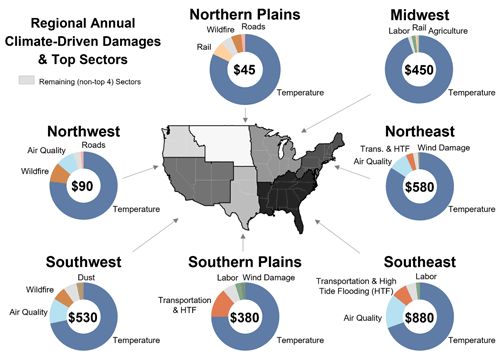
Figure A3Map of mean annual climate-driven damages for a subset of sectors across 10 000 projections in each of the seven US regions in the year 2090 (non-discounted). Damages are in billions of 2020 USD. Donut charts show the absolute damages (in billions) in each region for those sectors included in FrEDI and the top four sectors with the largest annual climate-driven damages. The share of damages from all remaining sectors are shown by the light gray wedge.
A1 Detailed results to 2090
FrEDI also has a module to incorporate information from the recent EPA Report of Climate Change and Social Vulnerability in the United States: A Focus on Six Impacts (EPA, 2021a) (hereafter called the SV Report) to assess the differential climate-driven impacts in 2090 across different socially vulnerable groups. As described in the SV Report, this analysis considers four categories for which there is evidence of differential vulnerability. These groups are listed in Table A2.
A2 FrEDI adaptation and uncertainty results
FrEDI also has the additional capability to investigate some of these adaptation options in select sectors by reflecting the treatment in the underlying sector studies. FrEDI maintains adaptation assumptions from the underlying studies that form the basis of FrEDI's temperature-driven sectoral damage functions. For most of these studies, because the implicit or explicit impact response function is calibrated to historical or current data, this means that historically practiced adaptation or hazard avoidance actions are “baked in” – but enhanced adaptation action or new (currently unknown) technologies are not considered. The exceptions include coastal property and select other infrastructure sectors, where the underlying studies consider specific adaptation actions. These have been incorporated into FrEDI. For example, for the coastal flooding sector, FrEDI's default adaptation assumption is a reactive adaptation scenario, as defined in Neumann et al. (2021), and includes the costs (and reflects the hazard reduction benefits) related to elevation of properties and armoring, where and when the benefits exceed the costs of this measure, and expanded beach nourishment at locations where it is currently practiced. No other measures are included. There is an option in FrEDI, however, for the user to select either a no adaptation scenario for this sector, which excludes the options above and measures that might hold back floodwaters, or a proactive adaptation scenario, where adaptation measures include elevation, beach nourishment, and armoring (either with bulkheads in protected areas or more expensive seawalls in areas exposed to higher open-ocean wave action) and are chosen based on the assumption that sea level will continue to rise in the future. It is difficult to comment on the realism of future action. There is some discussion in both Neumann et al. (2021) and Lorie et al. (2020), both of which make the point that even under current coastal hazards, cost-effective adaptation measures have not been adopted, probably because they involve short-term capital investment to yield future, uncertain benefits. This is one reason why proactive adaptation is not the default scenario in FrEDI.
For econometrically based sectors (e.g., labor), adaptation is included to the extent that adaptation is currently occurring (e.g., workplace safety procedures currently being utilized to protect against extreme temperatures; individual risk and damage avoidance behavior reflected in current practice). For infrastructure sectors (i.e., rail, roads, electricity transmission and distribution infrastructure; coastal properties; and transportation impacts from high-tide flooding), a no additional adaptation approach to infrastructure management does not incorporate climate change risks into the maintenance and repair decision-making process beyond baseline expectations and practice. The infrastructure sectors include two adaptation scenarios, following Melvin et al. (2017): reactive adaptation, where decision makers respond to climate change impacts by repairing damaged infrastructure but do not take actions to prevent or mitigate future climate change impacts (a variant on this scenario is the “reasonably anticipated adaptation” option for the high-tide flooding and traffic sector, which is defined similarly to the reactive scenario), and proactive adaptation, where decision makers take adaptive action with the goal of preventing infrastructure repair costs associated with future climate change impacts. This proactive adaptation scenario assumes well-timed infrastructure investments, which may be overly optimistic given that such investments have oftentimes been delayed and underfunded in the past and because decision makers and the public are typically not fully aware of potential climate risks (these barriers to realizing full deployment of cost-effective adaptation are described in Chambwera et al., 2014).
Table A3 shows that climate damages are sensitive to assumptions in the adaptation scenarios with mean 2090 annual damages of up to 2 to nearly 500 times larger in proactive or direct adaptation scenarios relative to damages when considering no adaptation. This illustrates adaptation has the capacity to both exacerbate and ameliorate future climate-driven damages.
Table A3Annual mean (and 95 % confidence interval) climate-driven damages in 2090 for sectors that include different adaptation options. Damages are in billions of dollars (2020 USD).
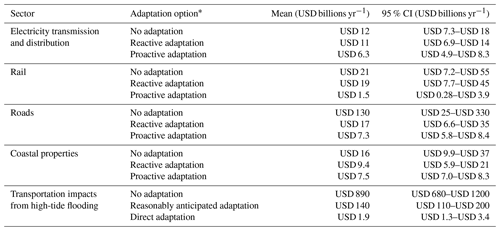
∗ The default adaptation assumption in FrEDI is the reactive or reasonably anticipated adaptation option.
In addition to adaptation scenarios, FrEDI also has the capability to explore the sensitivity of future climate damages to specific changes in additional sectors, including agricultural damages with and without CO2 fertilization, a lower air quality precursor emissions scenario, and high and low confidence intervals associated with damage functions specifically from temperature-related mortality. The Cromar et al. (2022) study also provides a standard error of the impact function's relative risk coefficient, which was used to develop a 90 % confidence interval around this parameter. The 90 % confidence interval supports the calculation of impacts for the low and high end of the confidence interval (5th and 95th percentile values) within FrEDI, as well as a central estimate that corresponds to the mean result. The Hsiang et al. (2017) study authors also shared results from uncertainty modeling in the underlying work, which was also used to develop a 90 % confidence interval of results. These uncertainty results support the calculation of the low and high end of the confidence interval (5th and 95th percentile values) within FrEDI, as well as a central estimate which corresponds to the median result (50th percentile).
There are currently three underlying temperature-related mortality studies within FrEDI. Table A4 provides a snapshot of the parametric uncertainty within each temperature-related mortality estimate, as well as structural damage function uncertainty, by comparing impacts across multiple studies. To separately evaluate the level of damage-function-related uncertainty compared to other sources of uncertainty presented in the main text (e.g., socioeconomics and climate), we show the mean damages from each damage function in Table A4, as calculated as the average across the RFF-SPs, as well as the 90th confidence intervals, as calculated by taking the average across the RFF-SPs for the damages projected by the high and low confidence interval damage functions. Compared to Table A1, Table A4 shows smaller projected ranges in temperature-related mortality damages than the ranges in damages derived from combined uncertainties in socioeconomic and climate parameters. We do not present these uncertainty levels in the main text as only a select number of sectors currently included with the FrEDI framework include information that allows us to evaluate parametric and structural damage function uncertainty. We also note that the underlying data in Hsiang et al. (2017) is calculated as the median, and therefore we are taking the mean across the RFF-SPs and the median damages. The Mills et al. (2014) study evaluates two scenarios, one with adaptation and one without adaptation.
Table A4Annual mean (90th % confidence interval) climate-driven damages in 2090 for premature mortality from temperature across three separate studies. Damages are in billions of dollars (2020 USD). Cromar et al. (2022) is used for temperature-related mortality throughout the analysis presented in the main text.
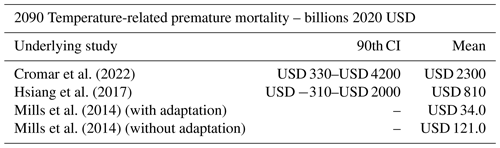
A3 FrEDI through 2300
FrEDI was calibrated to estimate impacts for detailed 21st century scenarios and trajectories, as described in Sarofim et al. (2021). Extending the FrEDI approach to 2300 requires two adjustments to adapt the sensitivity of the model to climate drivers and to socioeconomic conditions beyond the 21st century. First, we consider how the sensitivity to climate drivers (temperatures and inputs) might differ from 21st century conditions. FrEDI damages were originally calibrated for temperatures from 0 to 6∘ relative to the 1986 to 2005 era and SLR for 21st century trajectories that result in 30 to 250 cm global mean sea level (GMSL) rise outcomes by 2100. The original framework only returns physical and economic damage estimates within those bounds. In the modified FrEDI, damage estimates for temperature inputs above these bounds are calculated by extrapolating damages per degree using the change in damages between 5 and 6∘. SLR inputs above the bounds are extrapolated based on the damages per centimeter of SLR modeled by the two highest sea level scenarios in 2090.
Up to 6∘, FrEDI uses a piecewise linear function to estimate damages. This approach captures nonlinearities from the underlying impact models. However, for temperatures above the calibration regime, FrEDI assumes a linear rate of change in damages equal to the change in damages from 5 to 6∘. This assumption is likely to be conservative: Hsiang et al. (2017) found that combined damages in the United States increased quadratically with temperature, and Weitzman (2012) suggested that while a quadratic damage form might be reasonable for temperature changes up to 2.5 ∘C globally, for higher temperatures it would make sense for damages to increase more quickly, as standard damage functions are unlikely to capture the sheer magnitude of impacts resulting from the kind of dramatic changes the planet would undergo at temperature changes substantially higher than that.
Second, we consider how the sensitivity to socioeconomic drivers continues beyond 2090 through 2300 on a sector-specific basis (Table A5). Damage estimates in FrEDI reflect year-specific socioeconomic conditions. There are several ways these conditions are defined through 2090 and linked to the damage estimates for temperature-based damages. Treatment for 2090 through 2300 is explained after the description of the original definition for each category of adjustments.
Table A5Summary of the strategy for extending FrEDI sectoral results from 2090 to the 2300 modeling horizon. The impact column provides details for subcategories of impacts estimated within the framework. Wildfire sector subcategories include morbidity and mortality associated with air quality impacts and fire suppression response costs – these two classes of subcategories are listed separately because they employ different extension strategies.
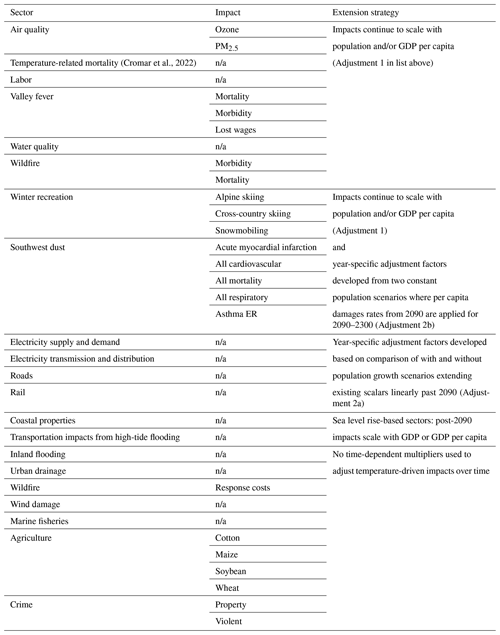
n/a: not applicable.
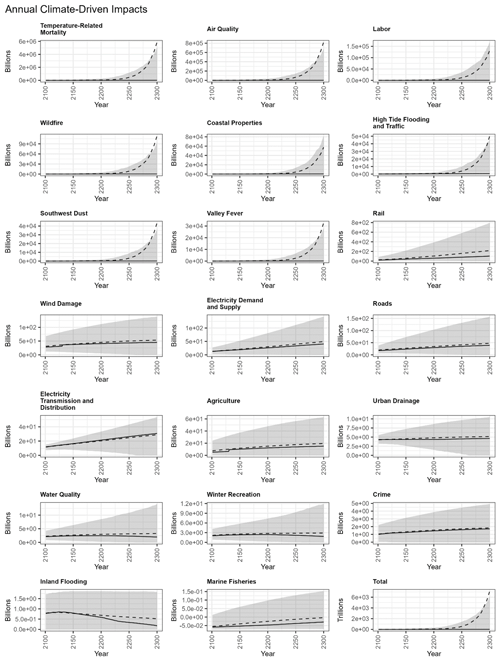
Figure A4Time series of sectoral damages across all 10 000 projections from 2100 to 2300 ordered by decreasing mean damages in the year 2300. The lower-right panel shows total damages summed across all sectors. The dashed (solid) line shows the mean (median) damages each year. Shaded areas show the 95 % CI. Annual damages are in units of billions of 2020 USD (trillions for the total panel).
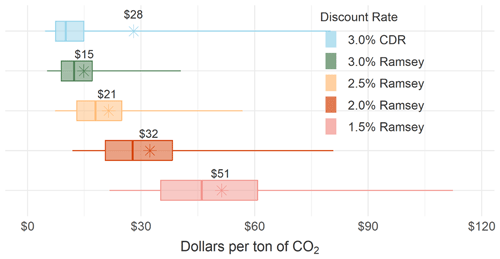
Figure A5Net present value of future damages from one tonne of CO2 for damages occurring only within the CONUS. Units are in dollars (2020 USD) per tonne of CO2 emitted. CDR refers to constant discount rate. Whiskers represent the 2.5th and 97.5th percentiles, while boxes span the 25th to 75th percentiles. Mean values (stars and text) along with median values (vertical lines) are also shown.
-
Impacts scale with population and/or GDP per capita. For sectors with explicit links to population and GDP, temperature-based damage estimates are scaled based on the population and GDP trajectory for a defined run. This is most common for health sectors, where total cases scale linearly with population and valuation of cases scales with GDP per capita. For example, willingness to pay to reduce fatality risk (referred to as the value of statistical life or VSL) is adjusted based on the projection of GDP per capita and a default income elasticity of 1.0. For 2090 through 2300, defined population and GDP trajectories continue to scale damage estimates through 2300.
-
Year-specific adjustment factors. In sectors where population and/or GDP per capita enter the impact function in complex ways that cannot be extracted and replicated within the FrEDI framework, a series of year-specific adjustment factors defined based on the underlying study are used to adjust damages over time and/or space. For example, changes in health outcomes over time driven by demographic composition (e.g., population by age group or geographic distribution within region, which affect baseline mortality rates or exposure) are incorporated in FrEDI as year-specific adjustment factors. These factors are derived from the underlying studies via two methods.
- a.
By comparing per capita damage rates from a constant population run to a run that incorporates population growth11, resulting in a time series of adjustment factors. For 2090 through 2300, the time series of adjustment factors is either linearly extrapolated through 2300 or held constant at 2090 levels based on the observed trends for 2010 through 2090 and the interpretation of the factor.
- b.
By comparing per capita damage rates for two constant population scenarios (i.e., 2010 and 2090) and interpolating for between years. For 2090 through 2300, per capita damage rate adjustments are held at 2090 levels through 2300.
No time-dependent adjustments. Some sectors – which, in general, make up a small portion of overall damages – are not adjusted for socioeconomic projections but vary based only on sensitivity to projected temperature (Table A5). For 2090 through 2300, no additional adjustments were necessary.
- a.
Some sectors utilize more than one method (e.g., Southwest dust outcomes scale linearly with population, as in method 1 in the list above, and per capita mortality rates are adjusted over time based on method 2a).
Sea level rise-based damages in FrEDI are derived from damages in the underlying studies that are year- and sea-level-rise-specific through 2100, thus no additional time-dependent adjustments are necessary for that time frame. Damages in each year reflect real property prices and adaptation decisions made in previous periods. For 2090 through 2300, damages post-2100 are based on sea-level-rise-based damages from 2100 adjusted for real property price appreciation using GDP per capita and an income elasticity of 0.45, consistent with the underlying Neumann et al. (2021) study.
As described in the main text, FrEDI is run through 2300 (Fig. A4) to calculate the net present damages associated with an additional pulse of 1 t of CO2 in the year 2020. In addition to the Ramsey discounting approach presented in the main text, Fig. A5 provides a comparison to the net present damages calculated using a constant discount rate of 3 %, consistent with OMB Circular A-4 (White House, 2003).
The Framework for Evaluating Damages and Impacts (FrEDI) is available on the U.S. EPA Enterprise GitHub https://doi.org/10.5281/zenodo.8211790 (McDuffie et al., 2023). FaIR is available at https://doi.org/10.5281/zenodo.1247898 (Smith et al., 2018b). The RFF SP projections are available at https://zenodo.org/record/6016583 (Rennert et al., 2022a), and the SSP projections are available at https://tntcat.iiasa.ac.at/SspDb/dsd?Action=htmlpage.
All code and data associated with this study are available at https://doi.org/10.5281/zenodo.8211790 (McDuffie et al., 2023).
CH, EEMD, and MS drafted the manuscript text and figures with contributions from all co-authors. BP, EEMD, KN, and CH conducted the computational analysis. KN and JW developed the FrEDI code. SB drafted Fig. 1 and provided input on graphics, and all authors contributed to the writing of the manuscript.
The contact author has declared that none of the authors has any competing interests.
Publisher's note: Copernicus Publications remains neutral with regard to jurisdictional claims in published maps and institutional affiliations.
The views presented in this paper are solely those of the authors and do not necessarily represent the views or policies of the U.S. Environmental Protection Agency. The authors also wish to acknowledge research assistance and other analytic support from William Maddock, Hayley Kunkle, Anthony Gardella, and Charles Fant.
Support for industrial economics was provided under EPA contract nos. 47QFSA21D0002 and 140D0420A0002.
This paper was edited by Christian Franzke and reviewed by two anonymous referees.
Armstrong McKay, D. I., Staal, A., Abrams, J. F., Winkelmann, R., Sakschewski, B., Loriani, S., Fetzer, I., Cornell, S. E., Rockström, J., and Lenton, T. M.: Exceeding 1.5 ∘C global warming could trigger multiple climate tipping points, Science, 377, eabn7950, https://doi.org/10.1126/science.abn7950, 2022.
Barron, A. R.: Time to refine key climate policy models, Nat. Clim. Change, 8, 350–352, https://doi.org/10.1038/s41558-018-0132-y, 2018.
Carleton, T. and Greenstone, M.: A Guide to Updating the US Government's Social Cost of Carbon, Rev. Environ. Econ. Policy, 16, 196–218, https://doi.org/10.1086/720988, 2022.
Carleton, T., Jina, A., Delgado, M., Greenstone, M., Houser, T., Hsiang, S., Hultgren, A., Kopp, R. E., McCusker, K. E., Nath, I., Rising, J., Rode, A., Seo, H. K., Viaene, A., Yuan, J., and Zhang, A. T.: Valuing the Global Mortality Consequences of Climate Change Accounting for Adaptation Costs and Benefits, Q. J. Econ., 137, 2037–2105, https://doi.org/10.1093/qje/qjac020, 2022.
Council of Economic Advisers (CEA): Discounting for public policy: Theory and recent evidence on the merits of updating the discount rate, Issue brief, Washington, DC: Executive Office of the President, 2017.
Cromar, K. R., Anenberg, S. C., Balmes, J. R., Fawcett, A. A., Ghazipura, M., Gohlke, J. M., Hashizume, M., Howard, P., Lavigne, E., Levy, K., Madrigano, J., Martinich, J. A., Mordecai, E. A., Rice, M. B., Saha, S., Scovronick, N. C., Sekercioglu, F., Svendsen, E. R., Zaitchik, B. F., and Ewart, G.: Global Health Impacts for Economic Models of Climate Change: A Systematic Review and Meta-Analysis, Ann. Am. Thorac. Soc., 19, 1203–1212, https://doi.org/10.1513/AnnalsATS.202110-1193OC, 2022.
Depsky, N., Bolliger, I., Allen, D., Choi, J. H., Delgado, M., Greenstone, M., Hamidi, A., Houser, T., Kopp, R. E., and Hsiang, S.: DSCIM-Coastal v1.0: An Open-Source Modeling Platform for Global Impacts of Sea Level Rise, EGUsphere [preprint], https://doi.org/10.5194/egusphere-2022-198, 2022.
EPA: Multi-Model Framework for Quantitative Sectoral Impacts Analysis: A Technical Report for the Fourth National Climate Assessment, U.S. Environmental Protection Agency, EPA 430-R-17-001, 2017a.
EPA: Updates To The Demographic And Spatial Allocation Models To Produce Integrated Climate And Land Use Scenarios (Iclus), EPA/600/R-16/366F, 2017b.
EPA: Climate Change and Social Vulnerability in the United States: A Focus on Six Impacts, U.S. Environmental Protection Agency, EPA 430-R-21-003, 2021a.
EPA: Technical Documentation on the Framework for Evaluating Damages and Impacts (FrEDI), U.S. Environmental Protection Agency, EPA 430-R-21-004, 2021b.
Forster, P., Storelvmo, T., Armour, K., Collins, W., Dufresne, J.-L., Frame, D., Lunt, D. J., et al.: The Earth’s Energy Budget, Climate Feedbacks, and Climate Sensitivity, in: Climate Change 2021: The Physical Science Basis. Contribution of Working Group I to the Sixth Assessment Report of the Intergovernmental Panel on Climate Change, edited by: Masson-Delmotte, V., Zhai, P., Pirani, A., Connors, S. L., Péan, C., Berger, S., Caud, N., Chen, Y., Goldfarb, L., Gomis, M. I., Huang, M., Leitzell, K., Lonnoy, E., Matthews, J. B. R., Maycock, T. K., Waterfield, T., Yelekçi, O., Yu, R., and Zhou, B., Cambridge University Press, Cambridge, United Kingdom and New York, NY, USA, 923–1054, https://doi.org/10.1017/9781009157896.009, 2021.
U.S. Government Accountability Office (GAO): Climate Change: Information on Potential Economic Effects Could Help Guide Federal Effort to Reduce Fiscal Exposure GAO-17-720. October, https://www.gao.gov/products/gao-17-720 (last access: 12 October 2022), 2017.
Heutel, G., Miller, N. H., and Molitor, D.: Adaptation and the Mortality Effects of Temperature across U.S. Climate Regions, Rev. Econ. Stat., 103, 740–753, 2021.
Hsiang, S., Kopp, R., Jina, A., Rising, J., Delgado, M., Mohan, S., Rasmussen, D. J., Muir-Wood, R., Wilson, P., Oppenheimer, M., Larsen, K., and Houser, T.: Estimating economic damage from climate change in the United States, Science, 356, 1362–1369, https://doi.org/10.1126/science.aal4369, 2017.
Hultgren, A., Carleton, T., Delgado, M., Gergel, D. R., Greenstone, M., Houser, T., Hsiang, S., Jina, A., Kopp, R. E., Malevich, S. B., McCusker, K. E., Mayer, T., Nath, I., Rising, J., Rode, A., and Yuan, J.: Estimating Global Impacts to Agriculture from Climate Change Accounting for Adaptation, https://doi.org/10.2139/ssrn.4222020, 2022.
Kopp, R. E., Kemp, A. C., Bittermann, K., Horton, B. P., Donnelly, J. P., Gehrels, W. R., Hay, C. C., Mitrovica, J. X., Morrow, E. D., and Rahmstorf, S.: Temperature-driven global sea-level variability in the Common Era, P. Natl. Acad. Sci. USA, 113, 1434–1441, https://doi.org/10.1073/pnas.1517056113, 2016.
Kotchen, M. J.: Which Social Cost of Carbon? A Theoretical Perspective, J. Assoc. Environ. Resour. Econ., 5, 673–694, https://doi.org/10.1086/697241, 2018.
Lay, C. R., Sarofim, M. C., Vodonos Zilberg, A., Mills, D. M., Jones, R. W., Schwartz, J., and Kinney, P. L.: City-level vulnerability to temperature-related mortality in the USA and future projections: a geographically clustered meta-regression, Lancet Planet. Health, 5, 338–346, https://doi.org/10.1016/S2542-5196(21)00058-9, 2021.
Lorie, M., Neumann, J. E., Sarofim, M. C., Jones, R., Horton, R. M., Kopp, R. E., Fant, C., Wobus, C., Martinich, J., O'Grady, M., and Gentile, L. E.: Modeling coastal flood risk and adaptation response under future climate conditions, Clim. Risk Manag., 29, 100233, https://doi.org/10.1016/j.crm.2020.100233, 2020.
Martinich, J. and Crimmins, A.: Climate damages and adaptation potential across diverse sectors of the United States, Nat. Clim. Change, 9, 397–404, https://doi.org/10.1038/s41558-019-0444-6, 2019.
Martinich, J., DeAngelo, B., Diaz, D., Ekwurzel, B., Franco, G., Frisch, C., McFarland, J., and O'Neill, B.: Reducing Risks Through Emissions Mitigation. In Impacts, Risks, and Adaptation in the United States: Fourth National Climate Assessment, Volume II, edited by: Reidmiller, D. R., Avery, C. W., Easterling, D. R., Kunkel, K. E., Lewis, K. L. M., Maycock, T. K., and Stewart, B. C., U.S. Global Change Research Program, Washington, DC, USA, 1346–1386, https://doi.org/10.7930/NCA4.2018.CH29, 2018.
McDuffie, E., Hartin, C., Parthum, B.: USEPA/FrEDI_NPD: Accepted Paper (Version v1), Zenodo [data set, code], https://doi.org/10.5281/zenodo.8211790, 2023.
Meinshausen, M., Nicholls, Z. R. J., Lewis, J., Gidden, M. J., Vogel, E., Freund, M., Beyerle, U., Gessner, C., Nauels, A., Bauer, N., Canadell, J. G., Daniel, J. S., John, A., Krummel, P. B., Luderer, G., Meinshausen, N., Montzka, S. A., Rayner, P. J., Reimann, S., Smith, S. J., van den Berg, M., Velders, G. J. M., Vollmer, M. K., and Wang, R. H. J.: The shared socio-economic pathway (SSP) greenhouse gas concentrations and their extensions to 2500, Geosci. Model Dev., 13, 3571–3605, https://doi.org/10.5194/gmd-13-3571-2020, 2020.
Melvin, A. M., Larsen, P., Boehlert, B., Neumann, J. E., Chinowsky, P., Espinet, X., Martinich, J., Baumann, M. S., Rennels, L., Bothner, A., Nicolsky, D. J., and Marchenko, S. S.: Climate change damages to Alaska public infrastructure and the economics of proactive adaptation, P. Natl. Acad. Sci. USA, 114, 122–131, https://doi.org/10.1073/pnas.1611056113, 2017.
National Academies of Sciences (NAS): Engineering, and Medicine, Valuing Climate Damages: Updating Estimation of the Social Cost of Carbon Dioxide. Washington, DC: The National Academies Press, https://doi.org/10.17226/24651, 2017.
Nordhaus, W. D.: Revisiting the social cost of carbon, P. Natl. Acad. Sci. USA, 114, 1518–1523, https://doi.org/10.1073/pnas.1609244114, 2017.
Office of Management and Budget (OMB): Circular A-4, Regulatory Analysis. OMB, https://obamawhitehouse.archives.gov/omb/circulars_a004_a-4/ (last access: 12 October 2022), 2003.
O'Neill, B. C., Kriegler, E., Ebi, K. L., Kemp-Benedict, E., Riahi, K., Rothman, D. S., van Ruijven, B. J., van Vuuren, D. P., Birkmann, J., Kok, K., Levy, M., and Solecki, W.: The roads ahead: Narratives for shared socioeconomic pathways describing world futures in the 21st century, Glob. Environ. Change, 42, 169–180, https://doi.org/10.1016/j.gloenvcha.2015.01.004, 2017.
Rennert, K., Prest, B. C., Pizer, W., Newell, R. G., Anthoff, D., Kingdon, C., Rennels, L., Cooke, R., Raftery, A. E., Sevcikova, Hana, and Errickson, F.: The Social Cost of Carbon: Advances in Long-Term Probabilistic Projections of Population, GDP, Emissions, and Discount Rates, Resour. Future, Working Paper 21–28, 2021.
Rennert, K., Prest, B. C., Pizer, W. A., Newell, R. G., Anthoff, D., Kingdon, C., Rennels, L., Cooke, R., Raftery, A. E., Ševčíková, H., and Errickson, F.: Resources for the Future Socioeconomic Projections (RFF-SPs) (Version 5), Zenodo [code], https://doi.org/10.5281/zenodo.6016583, 2022a.
Rennert, K., Errickson, F., Prest, B. C., Rennels, L., Newell, R. G., Pizer, W., Kingdon, C., Wingenroth, J., Cooke, R., Parthum, B., Smith, D., Cromar, K., Diaz, D., Moore, F. C., Müller, U. K., Plevin, R. J., Raftery, A. E., Ševèíková, H., Sheets, H., Stock, J. H., Tan, T., Watson, M., Wong, T. E., and Anthoff, D.: Comprehensive Evidence Implies a Higher Social Cost of CO2, Nature, 1–3, https://doi.org/10.1038/s41586-022-05224-9, 2022b.
Revesz, R., Greenstone, M., Hanemann, M., Livermore, M., Sterner, T., Grab, D., Howard, P., and Schwartz, J.: Best cost estimate of greenhouse gases, Science, 357, 655–655, https://doi.org/10.1126/science.aao4322, 2017.
Riahi, K., van Vuuren, D. P., Kriegler, E., Edmonds, J., O’Neill, B. C., Fujimori, S., Bauer, N., Calvin, K., Dellink, R., Fricko, O., et al.: The Shared Socioeconomic Pathways and their energy, land use, and greenhouse gas emissions implications: An overview, Global Environ. Change, 42, 153–168, https://doi.org/10.1016/j.gloenvcha.2016.05.009, 2017.
Rising, J. and Devineni, N.: Crop switching reduces agricultural losses from climate change in the United States by half under RCP 8.5, Nat. Commun., 11, 4991, https://doi.org/10.1038/s41467-020-18725-w, 2020.
Rising, J., Tedesco, M., Piontek, F., and Stainforth, D. A.: The missing risks of climate change, Nature, 610, 643–651, https://doi.org/10.1038/s41586-022-05243-6, 2022.
Rode, A., Carleton, T., Delgado, M., Greenstone, M., Houser, T., Hsiang, S., Hultgren, A., Jina, A., Kopp, R. E., McCusker, K. E., Nath, I., Rising, J., and Yuan, J.: Estimating a social cost of carbon for global energy consumption, Nature, 598, 308–314, https://doi.org/10.1038/s41586-021-03883-8, 2021.
Sarofim, M. C., Martinich, J., Neumann, J. E., Willwerth, J., Kerrich, Z., Kolian, M., Fant, C., and Hartin, C.: A temperature binning approach for multi-sector climate impact analysis, Clim. Change, 165, 22, https://doi.org/10.1007/s10584-021-03048-6, 2021.
Sarofim, M. C., Saha, S, Hawkins, M. D., Mills, D. M., Horton, R., Kinney, P., Schwartz, J., and St. Juliana, A.: Ch. 2: Temperature-Related Death and Illness. The Impacts of Climate Change on Human Health in the United States: A Scientific Assessment., U.S. Global Change Research Program, Washington, DC, 43–68, 2016.
Smith, C. J., Forster, P. M., Allen, M., Leach, N., Millar, R. J., Passerello, G. A., and Regayre, L. A.: FAIR v1.3: a simple emissions-based impulse response and carbon cycle model, Geosci. Model Dev., 11, 2273–2297, https://doi.org/10.5194/gmd-11-2273-2018, 2018a.
Smith, C., Millar, R., Nicholls, Z., Allen, M., Forster, P., Leach, N., Passerello, G., and Regayre, L.: FAIR – Finite Amplitude Impulse Response Model (multi-forcing version), in: Geoscientific Model Development (v1.3.2). Zenodo [code], https://doi.org/10.5281/zenodo.1247898, 2018b.
Weitzman, M.: GHG Targets as Insurance Against Catastrophic Climate Damages, J. Publ. Econ. Theory, 14, 221–244, 2012.
Wong, T. E., Bakker, A. M. R., Ruckert, K., Applegate, P., Slangen, A. B. A., and Keller, K.: BRICK v0.2, a simple, accessible, and transparent model framework for climate and regional sea-level projections, Geosci. Model Dev., 10, 2741–2760, https://doi.org/10.5194/gmd-10-2741-2017, 2017.
Wong, T. E., Rennels, L., Errickson, F., Srikrishnan, V., Bakker, A., Keller, K., and Anthoff, D.: MimiBRICK.jl: A Julia package for the BRICK model for sea-level change in the Mimi integrated modeling framework, J. Open Source Softw., 7, 4556, https://doi.org/10.21105/joss.04556, 2022.
Woodard, D. L., Shiklomanov, A. N., Kravitz, B., Hartin, C., and Bond-Lamberty, B.: A permafrost implementation in the simple carbon–climate model Hector v.2.3pf, Geosci. Model Dev., 14, 4751–4767, https://doi.org/10.5194/gmd-14-4751-2021, 2021.
https://github.com/OMS-NetZero/FAIR/releases/tag/v1.6.2 (last access: 8 July 2022)
See Rennert et al. (2022b) for more detail on the RFF-SPs and FaIR parameter sets. Each of the 10 000 RFF-SPs are assumed to be equally likely.
All dollar values in this paper are presented in 2020 US dollars. Any necessary transformations in the inputs (e.g., RFF-SPs are in 2011 USD, FrEDI takes in 2015 USD, and FrEDI results are presented in 2020 USD) are performed using the U.S. Bureau of Economic Analysis (BEA) national data on annual implicit price deflators for US GDP, the top row of BEACE3 Table 1.1.9.
This analysis uses the term BIPOC to refer to individuals identifying as Black or African American, American Indian or Alaska Native, Asian, Native Hawaiian or Other Pacific Islander, and/or Hispanic or Latino. It is acknowledged that there is no “one size fits all” language when it comes to talking about race and ethnicity and that no one term is going to be embraced by every member of a population or community. The use of BIPOC is intended to reinforce the fact that not all people of color have the same experience and cultural identity. This report therefore includes, where possible, results for individual racial and ethnic groups.
For Ramsey discounting calibrated to near-term target discount rates of 1.5 %, 2.5 %, or 3 %, ρ=0.01 %, 0.5 %, and 0.8 % and η=1.02, 1.42, and 1.57, respectively.
Consistent with Rennert et al. (2022b), we use a stochastic Ramsey discount factor to discount future climate-driven damages.
Data from https://fred.stlouisfed.org/series/FYGDP, percentage decline in annual GDP from 2008 to 2009.
https://tntcat.iiasa.ac.at/SspDb/dsd?Action=htmlpage&page=80, (last access: 5 October 2022)
Net present damages resulting from an additional tonne of CO2 emissions is sometimes characterized as a “domestic social cost of carbon”.
Figure A5 additionally compares these results to those using a constant discount rate of 3 % for a comparison with the historical approach in Circular A-4 (White House, 2003).
Another, less common method for calculating adjustment factors is to compare two runs with and without climate change, each with population growth, to baseline damages (e.g., no population growth and no climate change).