the Creative Commons Attribution 4.0 License.
the Creative Commons Attribution 4.0 License.
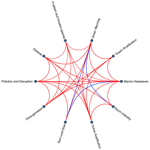
Considerations for determining warm-water coral reef tipping points
Paul Pearce-Kelly
Andrew H. Altieri
John F. Bruno
Christopher E. Cornwall
Melanie McField
Aarón Israel Muñiz-Castillo
Juan Rocha
Renee O. Setter
Charles Sheppard
Rosa Maria Roman-Cuesta
Chris Yesson
Warm-water coral reefs are facing unprecedented human-driven threats to their continued existence as biodiverse functional ecosystems upon which hundreds of millions of people rely. These impacts may drive coral ecosystems past critical thresholds, beyond which the system reorganises, often abruptly and potentially irreversibly; this is what the Intergovernmental Panel on Climate Change (IPCC, 2022) define as a tipping point. Determining tipping point thresholds for coral reef ecosystems requires a robust assessment of multiple stressors and their interactive effects. In this perspective piece, we draw upon the recent global tipping point revision initiative (Lenton et al., 2023a) and a literature search to identify and summarise the diverse range of interacting stressors that need to be considered for determining tipping point thresholds for warm-water coral reef ecosystems. Considering observed and projected stressor impacts, we endorse the global tipping point revision's conclusion of a global mean surface temperature (relative to pre-industrial) tipping point threshold of 1.2 °C (range 1–1.5 °C) and the long-term impacts of atmospheric CO2 concentrations above 350 ppm, while acknowledging that comprehensive assessment of stressors, including ocean warming response dynamics, overshoot, and cascading impacts, have yet to be sufficiently realised. These tipping point thresholds have already been exceeded, and therefore these systems are in an overshoot state and are reliant on policy actions to bring stressor levels back within tipping point limits. A fuller assessment of interacting stressors is likely to further lower the tipping point thresholds in most cases. Uncertainties around tipping points for such crucially important ecosystems underline the imperative of robust assessment and, in the case of knowledge gaps, employing a precautionary principle favouring lower-range tipping point values.
- Article
(658 KB) - Full-text XML
- BibTeX
- EndNote
Warm-water coral reefs (tropical and subtropical) support one-quarter to one-third of marine biodiversity (Plaisance et al., 2011), including over 25 % of marine fish species (Laffoley and Baxter, 2016). The estimated annual economic value of coral reef ecosystem services ranges from USD 29.8 billion (Cesar et al., 2003) up to USD 2.7 trillion (Souter et al., 2021), upon which at least 500 million people are reliant (IPBES, 2019). They are also among the most sensitive ecosystems to anthropogenic-driven stressors, with approximately 50 % of global live coral cover lost and accelerated declines seen over the last 30 years (IPBES, 2019), primarily due to ocean warming; but other factors have contributed locally such as fishing, pollution, disease, nutrient enrichment, and predation by crown-of-thorns starfish (IPCC, 2022). IPBES (2019) state that over 80 % of the world's coral reefs are severely overfished or have degraded habitats (McClanahan et al., 2015). Eddy et al. (2021) estimate that coral reef ecosystem services have halved since the 1950s. Although local stressors continue to impact coral reef health, climate-driven stressors have become the dominant threat to the functional viability of these ecosystems (IPBES, 2019; IPCC, 2022).
It is well established that coral reef ecosystems are vulnerable to multiple interacting tipping points (TPs) (Norström et al., 2016; Heinze et al., 2021; Armstrong-McKay et al., 2022; IPCC, 2022). The IPCC (2022) define a TP as “a critical threshold beyond which a system reorganises, often abruptly and/or irreversibly”. Coral reefs are prone to TPs that can produce coral die-offs and replacement by other ecological communities such as macroalgae, soft corals, or urchin barrens (Norström et al., 2016), with reductions in biodiversity and the degradation of ecosystem services (IPBES, 2019). Warm-water coral reefs cross a threshold of ecosystem collapse (Bland et al., 2018) when they cease to have the sufficient live coral cover (typically ∼10 %) necessary for supporting the wide diversity of taxa, ecological interactions, and a positive carbonate production state typical of a coral reef (Perry et al., 2013; Darling et al., 2019; Sheppard et al., 2020; Vercelloni et al., 2020; Armstrong-McKay et al., 2022). Coral mortality may take weeks or a few months for acute events (e.g. bleaching) or years for chronic threats (e.g. diseases), but prolonged failure to recover over a decade is necessary to qualify a coral reef as having “collapsed”.
Coral reef losses have accelerated in recent decades due to climate change and other stressors (IPBES, 2019; Souter et al., 2021), with high variability among regions, but some localised recovery and resilience have been observed (e.g. Richards et al., 2021). Localised responses of corals to increasing scales and intensities of stressors are aggregating at scales now exceeding 1000 km and manifesting as regional die-offs (e.g. western and central Indian Ocean, Great Barrier Reef, and the Mesoamerican Barrier Reef System) (Le Nohaïc et al., 2017; Muñiz-Castillo et al., 2019; Sheppard et al., 2020; Obura et al., 2022; Amir, 2022), with most regions experiencing multiple die-off events (Darling et al., 2019; Cramer et al., 2020; IPCC, 2022). Coral reef bleaching TPs have already been reached in seven ocean systems (IPCC, 2022).
Direct and indirect local human activities are increasingly degrading coral reef ecosystems through a combination of coastal development, water quality reduction, overharvesting, invasive species, and disease spread. At the local level, these stressors have already tipped some areas from coral- to macroalgae-dominated ecosystems (Bruno et al., 2009; IPBES, 2019; Souter et al., 2021). Local stressor impacts are increasingly exacerbated by anthropogenic climate change through, for example, a high abundance of macroalgae or urchins exacerbating coral loss after bleaching (Donovan et al., 2021).
It is important to consider the combined impact of multiple stressors. Doing so can significantly alter assessments of coral reef futures (Setter et al., 2022; Lenton et al., 2023a). Interactions between different stressors can be antagonistic (the combined effect is less than the additive), additive (the combined effect is equal to the sum of their individual effects), or synergistic (the combined effects exceed their individual effects) (see Fig. 2; Good and Bahr, 2021). Some studies find antagonistic interactions between multiple stressors (Darling et al., 2010; Johnson et al., 2022). However, a wide variety of synergistic interactions also occur (IPCC, 2022; Lenton et al., 2023a), generally lowering the thermal threshold for bleaching and/or mortality, accelerating collapse, or even surpassing thermal stress in local importance (Ban et al., 2013; Rocha et al., 2015; Anthony, 2016; Darling et al., 2019; IPBES, 2019; Cramer et al., 2020; Setter et al., 2022; Lenton et al., 2023a). The stressor onset rate can have a major effect on the significance, for example, of reef fish mortality (Genin et al., 2020). Depending on the onset rate and magnitude, the same interacting stressors may initially have antagonistic effects but may transition to additive or synergistic effects (e.g. Fisher et al., 2019).
Increasing atmospheric greenhouse gas (GHG) concentrations, especially carbon dioxide (CO2), are disrupting Earth's energy balance. The resultant Earth energy imbalance (EEI) is increasing atmospheric and ocean temperatures (IPCC, 2021; Loeb et al., 2021; Von Schuckmann et al., 2023). CO2 concentrations are the dominant drivers of the rate and magnitude of ocean warming and acidification (Meinshausen et al., 2020). Because of its large thermal inertia, the ocean takes hundreds of years to fully respond to the atmospheric temperature increases that human-driven GHG concentrations are causing (IPCC, 2021; Abraham et al., 2022; Cheng et al., 2022). The resultant “committed” heating and sea level rise (SLR) needs to be calculated for any given GHG/temperature level. Although ocean heat uptake and SLR take centuries to fully respond, it takes approximately 25–50 years for the majority of committed ocean warming to be realised (Hansen et al., 2005; Abrams et al., 2023), with the upper-ocean level having the shortest response time. Due to these inertia considerations, TP thresholds can be exceeded decades before the full physical impacts are observed.
Overshoot describes warming pathways that temporarily increase the global mean temperature over a specific temperature target (IPCC, 2022). An overshoot of multiple decades implies severe risks and irreversible impacts in many ecosystems (Meyer et al., 2022; Wunderling et al., 2023; Schleussener et al., 2024), including coral reefs from heat-related mortality and associated ecosystem transitions (high confidence) (IPCC, 2022). Overshoot is an urgent consideration for coral reefs because CO2 levels and global mean surface temperature have already exceeded critical thresholds; as such, we are already in overshoot, and this problem is compounded by stressor rate and magnitude (Lenton et al., 2023a).
TP cascades describe a TP in one system triggering, or stabilising, subsequent TPs in other systems (Rocha et al., 2018; Armstrong-McKay et al., 2022; IPCC, 2022; Wunderling et al., 2023). Here we summarise the most important stressors relevant to TP sensitivity for coral reefs and explore the interactions between them.
Warmer ocean temperatures, driven by anthropogenic climate change and compounded by El Niño–Southern Oscillation (ENSO) heating events, are the primary stressors of regional-scale and ocean-basin-scale mortality of scleractinian corals. Heat stress, in combination with irradiance, results from small increases in the seawater temperature above the summer maxima to which corals are acclimatised, destabilising the symbiosis between host corals and their symbiotic algae and commonly referred to as coral bleaching (Hughes et al., 2017; Houk et al., 2020; UNEP, 2020; IPCC, 2022).
Mass bleaching occurs when sea temperatures persist at more than 1 °C above established summer maxima for 8–12 weeks (known as 8–12 °C heating weeks or DHWs). Although mass bleaching has resulted in significant coral mortality, we note that with the loss of sensitive corals, acclimation, and adaptation, the definition of DHWs may require adjustment (Lenton et al., 2023a).
Previous assessments have highlighted the consequences of different levels of warming, as follows:
-
0.7 °C. “In the 1990s when global warming was around 0.7 °C large-scale coral reef bleaching also became apparent … supporting the lower boundary for this transition in respect of coral reefs” (IPCC, 2022).
-
1.0 °C. “[T]emperatures of just 1 °C above the long-term summer maximum … over 4–6 weeks are enough to cause mass coral bleaching … and mortality (very high confidence)” (Hoegh-Guldberg et al., 2018; Skirving et al., 2019).
-
1.2 °C. “Warm water (tropical) coral reefs are projected to reach a very high risk of impact at 1.2 °C …, with most available evidence suggesting that coral-dominated ecosystems will be non-existent at this temperature or higher (high confidence). At this point, coral abundance will be near zero at many locations and storms will contribute to `flattening' the three-dimensional structure of reefs without recovery, as already observed for some coral reefs (Alvarez-Filip et al., 2009)” (Hoegh-Guldberg et al., 2018). Coral reef bleaching TPs have already been passed in seven ocean systems (IPCC, 2022; Lenton et al., 2023a).
-
1.5 °C. “[C]oral reefs… will undergo irreversible phase shifts due to marine heatwaves with global warming levels >1.5 °C and are at high risk this century even in <1.5 °C scenarios that include periods of temperature overshoot beyond 1.5 °C (high confidence)” (IPCC, 2022). Projections predict 70 %–90 % coral loss at 1.5 °C (Hoegh-Guldberg et al., 2018; IPBES, 2019; Souter et al., 2021; Armstrong-McKay et al., 2022), whereas finer-scale modelling projects a 95 %–98 % loss (Kalmus et al., 2022) and suggests a 99 % loss (Dixon et al., 2022).
-
2.0 °C. “[L]iterature since AR5 has provided a closer focus on the comparative levels of risk to coral reefs at 1.5 °C versus 2 °C of global warming … reaching 2 °C will increase the frequency of mass coral bleaching and mortality to a point at which it will result in the total loss of coral reefs from the world's tropical and subtropical regions” (IPCC, 2018). Predictions show a 99 % coral loss at 2.0 °C (Frieler et al., 2013; Hoegh-Guldberg et al., 2018; IPBES, 2019; Knowlton et al., 2021; Souter et al., 2021; Armstrong-McKay et al., 2022; Wang et al., 2023). Finer-scale modelling projects a 100 % loss at 2.0 °C (Dixon et al., 2022; Kalmus et al., 2022).
Since the first global bleaching event of 1998, up to 71 % of the world's reefs have experienced three further global mass bleaching events, with a fourth event being experienced in 2023–2024 (https://www.noaa.gov/news-release/noaa-confirms-4th-global-coral-bleaching-event, last access: 17 January 2025).
Assessments of risk to corals from heating typically do not consider co-occurring or interacting stressors or the delayed heating response to atmospheric greenhouse gas concentrations. Ocean warming inertia may mask the impact severity of the stated greenhouse gas and temperature levels. When emission-driven temperature overshoot is considered, lower target temperatures can have similar impacts to higher ones, with little difference in the coral survival between an overshoot scenario that peaks at 2 °C and subsequently reduces in temperature to 1.5 °C versus a 2 °C scenario without a subsequent reduction in temperature (Tachiiri et al., 2019).
Tanaka and Van Houtan (2022) confirm the normalisation of extreme heating events. The frequency and duration of bleaching events are likely to increase, occurring earlier in the year and potentially overlapping with critical spawning periods (Mellin et al., 2024). The compounding heat stress of El Niño events (Claar et al., 2018; Hughes et al., 2018b; Lough et al., 2018) may increase with projected Arctic and Antarctic sea ice loss (England et al., 2020; Liu et al., 2022). Real-world observations from the NOAA Coral Reef Watch programme demonstrate that coral reef damage is accelerating and underscore the threat that anthropogenic climate change poses for the irreversible transformation of these essential ecosystems (Eakin et al., 2022).
Interactions of ocean warming and heatwaves with other stressors
Warming effects are so far-reaching in their impacts that they can adversely impact many other coral stressors. These stressors, in turn, can increase vulnerability to thermal stress. For example, heating-induced bleaching increases disease risk and lowers calcification, which increases the impact of ocean acidification (Davis et al., 2021; Burke et al., 2023). Corals that survive bleaching can have compromised growth rates and reproduction (Rodrigues and Padilla-Gamino, 2022; Speare et al., 2022; Briggs et al., 2024). Furthermore, warming oceans and heatwaves increase storm intensity and raise sea-level through thermal expansion and cryosphere melting.
Ocean stratification is the layering of water masses based on density. Stratified water layers are a barrier to mixing, which impacts the exchange of heat, oxygen, nutrients, and carbon between shallow and deep water. This impacts marine organisms in a number of significant ways, including changing primary productivity and potentially the entire marine food chain. Stratification has increased globally by 5.3 % in recent decades (Li et al., 2020).
Interactions
Stratification is strongly linked with warming oceans. Stratification magnifies the warming effect at the upper layers, thus increasing thermal stress to warm-water reefs. This is a vicious circle as warming oceans further increase stratification. Additionally, stratification reduces CO2 uptake, further exacerbating anthropogenic warming. Stratification impedes ocean mixing and impacts nutrient flows. Stratification is strongly linked with deoxygenation. Stratification is also linked with the melting of Antarctic ice shelves and sea level rise (Reed and Harrison, 2016; Li et al., 2020; Auger et al., 2021). Stratification is increasing, which has dramatic consequences for sea temperatures and CO2 concentrations (Goreau and Hayes, 2024)
Ocean acidification (OA) is the process of the increasing absorption of atmospheric CO2 by the surface seawaters of the oceans (Raven et al., 2005), which in turn reduces the calcification rates of most scleractinian tropical and subtropical corals (Comeau et al., 2014; Kornder et al., 2018) and can alter the photo-physiology and calcification physiology of some corals (Comeau et al., 2018). OA causes a change in the speciation of dissolved inorganic carbon and an increase in protons (Caldeira and Wickett, 2003; Feely et al., 2004; Sabine et al., 2004; Raven et al., 2005). This results in an increased dissolution of exposed calcareous material due to a decreased saturation state of CaCO3 and also the inhibition of calcification through increasing proton concentration with the calcifying space in corals and calcareous algae (Comeau et al., 2018, 2019).
OA causes declines in coral calcification rates (Comeau et al., 2018). Early work predicted a large-scale loss of coral calcification at catastrophic levels, whereby OA was projected to result in coral bleaching and, in some cases, net dissolution of corals (Leung et al., 2022). Contemporary research demonstrates that some corals are resistant to OA (Comeau et al., 2018; Kornder et al., 2018). The most comprehensive modelling estimates are that by the year 2100, coral calcification would decline by 1 % under RCP2.6, 4 % under RCP4.5, and 15 % under RCP8.5 (Cornwall et al., 2021). When combined solely with the metabolic effects of temperature increases, this decline would be 1 % (RCP2.6), 8 % (RCP4.5), and 33 % (RCP8.5). However, the calcification rates of susceptible coral taxa (e.g. Acropora spp.) would decline by much more, and resistant species (e.g. Pocillopora spp. or Porites spp. generally) could be unaffected.
The direct metabolic impacts of OA do not manifest a TP, but TPs at ecological levels are likely. Recent evidence indicates that ecological TPs within coral reefs caused solely by ocean acidification would occur around 550 ppm, roughly the same concentration of atmospheric CO2 that would cause detectable declines in both coral and coralline algal calcification (Cornwall et al., 2024). However, ecosystem trajectories are uncertain, and much more future research is required to determine the generality of these findings.
The adverse impacts on coral and coralline algal calcification are direct negative effects when combined with the direct positive effects on other taxa (such as opportunistic turfing algae). Susceptible species would start to give way to tolerant species over time as generally occurs at natural analogues in the field (Fabricius et al., 2011; Comeau et al., 2022), and other non-coral taxa would start to dominate space on what once were traditional coral reefs. Species that are capable of maintaining stable internal carbonate chemistry, or compensating for these changes, tend to be more resilient in the presence of OA.
Interactions
Reduced calcification increases disease risk, and weakened skeletons are vulnerable to storms (Suwa et al., 2010; Anthony et al., 2011; Setter et al., 2022). There is also some evidence that elevated CO2 will exacerbate heat-stress-induced declines in coral calcification and physiological performance, though the strength and direction of these interactions varies widely by coral reef taxa and even within different coral genera (Kornder et al., 2018). However, of greater immediate importance to the majority of corals will be successive marine heatwaves that will reduce the coral cover of less heat-tolerant species, populations, and genotypes over the majority of the oceans in the near future (van Hooidonk et al., 2014; Cornwall et al., 2021, 2023; Logan et al., 2021). Survivors of this human-driven evolutionary force will not necessarily be those that are resilient in the presence of OA, and thus numerous TPs could occur.
Deoxygenation on coral reefs is perhaps the least studied of the major threats directly linked to climate change (such as warming and acidification; Hughes et al., 2020). However, there is sufficient evidence to say that dissolved oxygen is a critical resource on coral reefs and that oxygen limitation (i.e. hypoxia) results in non-linearities and feedbacks that contribute to ecological tipping points (Nelson and Altieri, 2019). The consequences of crossing these TPs are perhaps most dramatically evident in sudden mass mortality events, which has led to calls to accelerate research on the deoxygenation on coral reefs (Altieri et al., 2017). The oxygen concentration threshold at which corals lose their ability to maintain homeostasis is 2 mg L−1, with lethal doses between 0.5–2 mg L−1 (Hughes et al., 2020; Johnson et al., 2021b). Previous mass extinctions have been linked to deoxygenation, indicating the potential severity of this threat (Liu et al., 2019).
The problem of deoxygenation on coral reefs is becoming more prevalent and severe in the Anthropocene from a combination of global climate change (Altieri and Gedan, 2015; Pezner et al., 2023) and local pollution in the form of excess nutrients and organic matter (Diaz and Rosenberg, 2008), which are magnified by local oceanographic patterns (Adelson et al., 2022). Around 13 % of coral reefs are at risk of deoxygenation, and this is likely to increase with continued climate change (Altieri et al., 2017; Pezner et al., 2023).
We suggest that evidence to date for feedbacks and non-linear thresholds indicates that a TP framework should be used to guide future research on deoxygenation on coral reefs and that hypoxia should be considered in studies of thermal stress and acidification.
Interactions
Climate-related variables of temperature and acidification are also likely to exacerbate deoxygenation by affecting the physiological responses of corals and other reef organisms. It is widely recognised that increased temperatures lead to increased metabolic demand and decreased tolerance thresholds in marine organisms, including corals (Vaquer-Sunyer and Duarte, 2011; Alderdice et al., 2022; Weber et al., 2012). Considering the co-occurrence and synergistic effects of these co-stressors with deoxygenation, a multi-stressor perspective is essential, and many of the assumed thresholds for TPs on coral reefs based on single- or even double-stressor treatments under laboratory experiments are likely to be overly conservative estimates. Coral reefs are vulnerable to a number of feedbacks that exacerbate deoxygenation events. These include bleaching (Altieri et al., 2017, 2021; Johnson et al., 2021a, b), excessive dead material from mass mortality events (Simpson et al., 1993), coral disease and algal growth (Dinsdale and Rohwer, 2011), and shifts in the coral microbiome (Howard et al., 2023).
The direct force of wind and waves, along with changes in storm direction, increases risks of physical damage and exposure to reduced water quality and sediment runoff (IPCC, 2018). Storms contribute to unstable rubble substrates, compromising coral settlement (Sheppard et al., 2020). Furthermore, frequent intense storms can hinder reef recovery (Puotinen et al., 2020). Setter et al. (2022) ascribe a threshold value of storm strength category <4 with a return time of >5 years.
Interactions
Ocean warming may increase the severity of cyclones (IPCC, 2021; Setter et al., 2022), and coral bleaching has likely reduced the ability of reefs to recover from cyclone damage (Laffoley and Baxter, 2016). The likelihood of more intense cyclones within time frames of coral recovery by mid-century poses a global threat to coral reefs and dependent societies (Cheal et al., 2017).
Storms can have an antagonistic interaction with heat stress, reducing bleaching severity, but also generate sediment resuspension (Gardner et al., 2005; Manzello et al., 2007; Carrigan and Puotinen, 2014; Puotinen et al., 2020; Setter et al., 2022). Reduced calcification increases susceptibility to storm impacts (Suwa et al., 2010; Anthony et al., 2011; Setter et al., 2022).
Sea level rise (SLR) can cause “reef drowning” from exceeding Darwin Point thresholds (Grigg, 2008). Saunders et al. (2016) note that while individual corals may keep pace with SLR, the likely maximum reef framework accretion rate on reef flats is only 3 mm yr−1. Saintilan et al. (2023) estimate likely vulnerability to relative SLR at 7 mm yr−1 for coral reef islands. Global mean sea level between 2006 and 2018 increased to 3.7 mm yr−1 (IPCC, 2021). Under SSP1-2.6, due to the risk of loss of reef structural integrity and transitioning to net erosion by mid-century, the rate of sea level rise is very likely to exceed that of reef growth by 2050, without adaptation (IPCC, 2022). Depending on the reef type and location, suggested SLR threshold rates range from 4–9 mm yr−1.
Closely connected seagrass and mangrove ecosystems (Guannel et al., 2016) are very vulnerable to projected SLR (Saunders et al., 2014; Törnqvist et al., 2021; Saintilan et al., 2023), which will further compromise coral reef resilience and functionality. In summary, SLR rate and magnitude look increasingly likely to overwhelm the accretion ability of coral reefs, which will be further challenged by increased wave energy, sedimentation, turbidity, and resultant compromised light conditions for symbiont photosynthesis (Saunders et al., 2014; Woodroffe and Webster, 2014; Törnqvist et al., 2021; Saintilan et al., 2023).
Interactions
Moderate rates of sea level rise may potentially provide cooling for some reefs contending with thermal stress and thus have an antagonistic effect (Baldock et al., 2014; Cinner et al., 2015; Brown et al., 2019; Zuo et al., 2021). However, the SLR rate and magnitude predictions (e.g. Ciraci et al., 2023; Vernimmen and Hooijer, 2023) imply increasingly synergistic impacts, especially in the tropics (Spada et al., 2013; Hooijer and Vernimmen, 2021). High SLR rate and magnitude can change the interactions from antagonistic to synergistic, for example, reducing light availability and increasing sedimentation and turbidity (Laffoley and Baxter, 2016; Perry et al., 2018; IPCC, 2022).
Here we use pollution as an all-encompassing term covering sediment, eutrophication, turbidity, and chemicals, while disruption is a term covering local land use change, human population density, and overfishing. Sedimentation reduces water clarity and hence solar energy supply. Furthermore, sediments settling on corals require greater energy to be removed. Sedimentation is caused mainly by land-based activities such as coastal urbanisation, with plumes in large tropical river systems travelling many kilometres (Brodie et al., 2012). Organic pollution from sewage and agricultural run-off (e.g. fertiliser) are the main causes of eutrophication (increased nutrient content in water), which reduces light, poisons invertebrates, introduces pathogens, and reduces resistance to disease. This has direct impacts on corals including decreased colony sizes, growth anomalies, and reduced growth and survival (Setter et al., 2022). Metals and organic chemicals can rupture cell membranes and disrupt enzyme pathways, reducing the corals' ability to resist other stressors. Plastics have also been identified as a major cause of coral reef stress due to light interference, toxin release, physical damage, anoxia, and increasing the likelihood of pathogen disease 20-fold (Lamb et al., 2018). Land use can be used as a proxy for quantifying land-based pollution and other human stressors on coral reefs (Packet et al., 2009; Cinner et al., 2012; Setter et al., 2022). Setter et al. (2022) use human population density as the closest indicator available to quantify local human stressors involving coral growth anomalies and disease, low biodiversity, fish biomass, and reduced growth and survival. To calculate the reef change threshold exceedance, Setter et al. (2022) use an ideal value of the summed proportion of agricultural/urban land use <0.5 in a 50 km radius around a reef. Perhaps the most direct disruptive impact is overfishing, with IPBES (2019) stating that more than 80 % of the world's coral reefs are severely overfished or have degraded habitats (McClanahan et al., 2015).
Interactions
Under certain circumstances, poor water quality can mediate bleaching resilience through a shading effect. Pollution exacerbates stress and increases disease risk, both of which are exacerbated by thermal stress. Eutrophication increases deoxygenation and exacerbates crown-of-thorns starfish (COTS) outbreaks (De'ath and Fabricius, 2010; Redding et al., 2013; Laffoley and Baxter, 2019; MacNeil et al., 2019), while overfishing is also linked to COTS outbreaks (Babcock et al., 2016). Sites with historic disturbance may recover more slowly from heat stress and storms (Walker et al., 2024). Overfishing can lead to algae overgrowth inducing disease and lowering calcification (Fabricius, 2005; Packett et al., 2009; Maina et al., 2013; Kroon et al., 2014; Prouty et al., 2017).
Diseases can be major drivers of the deterioration of coral reefs and are linked to major declines in coral abundance, reef functionality, and ecosystem services (Alvarez-Filip et al., 2022). Disease outbreaks have severe consequences for coral reef ecosystems, resulting in extensive coral mortality and endangering long-term survival. Noteworthy events include the rapid proliferation of diseases like stony coral tissue loss disease (SCTLD), black band disease, and various forms of white syndrome (Alvarez-Filip et al., 2022). Coral diseases are driven largely by a changing environment and are contributing to whole ecosystem regime shifts (Thurber et al., 2020). Although diseases are becoming increasingly prevalent with temperature rise and pollution, these, by themselves, have had relatively little overall impact outside of the Caribbean Sea to date. In the Caribbean, SCTLD is a major present source of coral mortality, impacting more than a third of all reef-building coral species present and potentially increasing the extinction risk of the Pillar coral Dendrogyra cylindrus (among others). The relative impact of diseases elsewhere is likely to change in the future, becoming more prevalent and interacting with heatwaves and other stressors (Estrada-Saldívar et al., 2021; Cavada-Blanco et al., 2022).
Interactions
Some coral diseases have been linked to marine heatwaves and the longer-term warming trend (Bruno et al., 2007; Randall and van Woesik, 2015). For example, viral infections of coral symbiotic dinoflagellate partners (Symbiodiniaceae) will likely increase as ocean temperatures continue to rise, potentially impacting the foundational symbiosis underpinning coral reef ecosystems (Howe-Kerr et al., 2023). Furthermore, predation scars leave corals susceptible to disease (Nicolet et al., 2018). Invasive species can directly cause or increase the risk of disease spread.
Increased native and invasive coral predators and competitors can have severe impacts on reefs. One example is the impact of COTS on the Great Barrier Reef (Uthicke et al., 2015). The coral-killing sponge, Terpios hoshinota, is a global invasive species which has led to a significant decline in living coral cover at various geographical locations (Thinesh et al., 2017).
Interactions
Warming is a driving factor in the increased impact of invasive and problem species. Studies on Mexican Pacific coast coral reefs confirmed that post-bleached corals are increasingly vulnerable to boring sponge impacts (Carballoe et al., 2012). COTS outbreaks appear to be significantly influenced by a combination of heat stress resiliency (Byrne et al., 2023) and increased larval survivorship due to higher food availability, linked with anthropogenic runoff and warmer sea temperature facilitating the faster settlement of larvae (Uthicke et al., 2015). Predation scars can leave corals susceptible to disease (Nicolet et al., 2018).
Chagos Archipelago demonstrates positive feedback (TPs)
Observations from the Chagos Archipelago, central Indian Ocean, reveal several related lessons. Coral cover collapsed by 90 % after heatwaves in 2015–2016 (Fig. 1). Very few adults capable of spawning survived, with new growth not observed for 3 years (Sheppard and Sheppard, 2019).
Settlement of larvae, when it occurred, was compromised by disintegrating substrates. In many shallow areas, where wave energy had already swept the substrate clear of rubble, large areas are becoming covered by the encrusting and bioeroding sponge Cliona spp. (Sheppard et al., 2020), and almost no coral settlers were seen in these areas. These sponges are clearly increasing, with one reef showing over 80 % Cliona cover preventing coral larvae settlement.
On at least one lagoon floor, the former foliaceous coral dominance was also killed, with skeletons disintegrating and resulting in fine sediment covering all surfaces. Sedimented surfaces and turbid water are not preferred conditions for larval settlement, with no juvenile corals recorded in such areas over many hectares.
The scenario of fewer corals producing fewer larvae, more turbid water in some areas, and less substrate available for settlement is a classical positive feedback or TP situation. These factors all act synergistically in a direction that inevitably leads to an increasingly impoverished reef system. Recovery from this will require a prolonged period without heat stress and a gradual removal of the vast volumes of sediment and rubble left from previous bleaching events (Sheppard and Sheppard, 2019).
The cascading effects of well-researched impacts in other globally important systems have not been sufficiently assessed for their potential impact on coral reef systems. Accelerating West Antarctic Ice Sheet melt (Naughten et al., 2023), increasing methane emissions (Zhang et al., 2023), and Arctic sea ice decline have the potential to increase the rate and magnitude of coral reef stressor impacts, including temperature and SLR. For example, Liu et al. (2022) predict that 37 %–48 % of the increase in strong El Niño events near the end of the 21st century is associated specifically with Arctic sea ice loss. Many climate impact predictions make assumptions about the stability of the wider Earth system, but this may not hold and lead to significant cascading impacts. For example, Ke et al. (2024) show a dramatic decline in land carbon sinks in 2023 which, if continued, will have wider implications on CO2 levels and associated stressors.
Lenton et al. (2023a) state that
The potential for coral adaptation to warming is a critical but poorly known factor, and subject to high levels of variation locally. The potential effectiveness of restoration for coral reefs at scale, and with enhanced capacity to resist future threats, are both currently poor. The effect of climate migration on coral recovery is not known, with potentially positive effects at higher latitude (with in-migration), but negative at lower latitudes (with out-migration, but no replacement; Herbert-Read et al., 2022).
The IPCC AR6 Impacts, Adaptation, and Vulnerability report states that “impacts of climate change may overwhelm attempts at restoration/conservation, particularly when the ecosystem is already near its TP, as is the case with tropical coral reefs (Bates et al., 2019; Bruno et al., 2019)”.
Mass coral mortality events repeated more than twice per decade and over local, regional, and ocean scales, and by aggregation to global scales, are increasingly recognised as giving insufficient time for the recovery of impacted populations and ecological function (Hughes et al., 2018a, b; Obura et al., 2022; Lenton et al., 2023a; Venegas et al., 2023). Ecological and biogeographical (spatial) feedback loops prevent recovery through the failure of reproduction, dispersal, recruitment, and growth of corals (Sheppard et al., 2020). Other stressors reduce the ability of corals to resist thermal stress, thus lowering tipping thresholds. Increasing the frequency and intensity of regional-scale coral mortality events (1+ °C warming) are suggestive of the majority of coral reefs already having reached their bleaching TP (IPCC, 2022). The potential for thermal refuges for corals under likely future scenarios is doubtful (Beyer et al., 2018; Dixon et al., 2022; Setter et al., 2022; Lenton et al., 2023a) as very few or no reef areas are predicted to remain below tipping thresholds of all key stressors. The existence of putative refuges at greater depths (Bongaerts and Smith, 2019) or higher latitudes (Setter et al., 2022) is not strongly supported by recent research (Hoegh-Guldberg et al., 2017, 2018; Rocha et al., 2018; Montgomery et al., 2021; IPCC, 2022).
There is evidence of the persistence of heat-adapted genotypes in some species, but the loss of poorly adapted corals leads to a loss of diversity (Fox et al., 2021). Although the potential for adaptation exists, stronger warming rates may outpace adaptive processes and limit coral persistence (Logan et al., 2021; Venegas et al., 2023). Historical and palaeo-evidence suggest fringe distributions are likely to be compromised by increasing frequency and intensity of extreme weather (Toth et al., 2021). Donovan et al. (2021) show that local stressors act synergistically with climate change to kill corals. Local factors such as a high abundance of macroalgae or urchins have magnified coral loss in the year after bleaching. Notably, the combined effects of increasing heat stress and macroalgae intensified coral loss, suggesting that effective local management, alongside global efforts to mitigate climate change, could aid coral survival. Agostini et al. (2021) suggest that ocean acidification will reshape coral communities around the world, selecting species that have an inherent resistance to elevated pCO2.
Kleypas et al. (2021) provide a blueprint for coral reef survival and state that existing conservation measures such as marine protected areas and fisheries management are no longer sufficient to sustain reef ecosystems, indicating a need for many additional and innovative actions to increase reef resilience. Anthony et al. (2020) discuss new interventions and provide a conceptual model to guide effective strategy choices. They also state that warm-adapted coral traits may not spread fast enough in most coral species to keep up with the rate of warming, even under strong carbon mitigation. Hughes et al. (2023) provide recommendations and a conceptual framework to guide restoration projects and state that coral restoration is likely to continue to fail unless climate change and other anthropogenic impacts are urgently reduced.
Robust inclusion of multiple interacting stressors into vulnerability assessments will lead to a greater understanding of coral reef futures and address concerns that assessments have been too reliant on temperature thresholds (McClanahan, 2022; Klein et al., 2024). Stressor onset rate, magnitude, and overshoot factors are important considerations for determining stressor interactions and their significance.
Veron et al. (2009) argue that to ensure the long-term viability of coral reefs, atmospheric CO2 levels must be reduced significantly below 350 ppm. Lenton et al. (2023a) recognise the long-term consequences of >350 ppm as a critical TP threshold, along with a global mean surface temperature (relative to pre-industrial) threshold of 1.2 °C (range 1–1.5 °C), while acknowledging that the “combined effects of long-term warming, sea level rise, ocean acidification, deoxygenation, and other stressors, bears more investigation”. The significance of both these TP thresholds is highlighted by the fact that global warming has already reached 1.2 °C, and CO2 levels have exceeded 420 ppm. Considering the calculations of von Schuckmann et al. (2020) that CO2 levels would need to be reduced to 353 ppm to realise the Paris temperature target, 350 ppm is likely to be insufficient for realising a 1.2 °C TP threshold, especially as other significant greenhouse gases are still increasing.
We note that interacting stressors, ocean response dynamics, GHG emissions overshoot, and cascade considerations have yet to be sufficiently evaluated. These and other uncertainties around TP sensitivities for such a crucially important ecosystem underline the imperative of robust assessment (Aronson and Precht, 2016; Dixon et al., 2021; Heinze et al., 2021; Laffoley et al., 2022; Lenton et al., 2023a) and, in the case of knowledge gaps and uncertainties, employing a precautionary principle (Rockström et al., 2021; OECD, 2022; Deutloff et al., 2023; Lenton et al., 2023b; Ripple et al., 2023; Fletcher et al., 2024) favouring lower-range threshold values. Recognising threat severity is essential if the necessary response actions are to be realised.
No data sets were used in this article.
PPK conceptualized the paper. PPK and CY prepared the majority of the original draft. CC prepared the original draft of the ocean acidification section. CS prepared the original draft of the case study. AA prepared the original draft of the deoxygenation section. All authors reviewed and edited the text.
The contact author has declared that none of the authors has any competing interests.
Publisher's note: Copernicus Publications remains neutral with regard to jurisdictional claims made in the text, published maps, institutional affiliations, or any other geographical representation in this paper. While Copernicus Publications makes every effort to include appropriate place names, the final responsibility lies with the authors.
This article is part of the special issue “Tipping points in the Anthropocene”. It is a result of the “Tipping Points: From Climate Crisis to Positive Transformation” international conference hosted by the Global Systems Institute (GSI) and University of Exeter (12–14 September 2022), as well as the associated creation of a Tipping Points Research Alliance by GSI and the Potsdam Institute for Climate Research, Exeter, Great Britain, 12–14 September 2022.
We are grateful to Richard Betts for advice on atmospheric CO2 and temperature associations and ocean warming response times, and we would also like to thank Katja Frieler, Malte Meinshausen, and Ove Hoegh-Guldberg. We thank our reviewers (including Lyndon DeVantier) for their valuable and constructive comments. We would like to express our condolences regarding the loss of our fellow author, Charles Sheppard, who sadly passed away during the production of this work, and recognise his remarkable contributions to coral biology and conservation.
Andrew H. Altieri has been supported by the U.S. National Science Foundation through their division of Ocean Sciences under grant no. OCE-2238422. Christopher E. Cornwall has been supported by Coastal People Southern Skies Centre of Research Excellence. Rosa Roman received support from the BNP PARIBAS Foundation for the CORESCAM project on coastal resilience to extreme events in the Caribbean and central America. Chris Yesson has been supported by funding from Research England.
This paper was edited by Gabriele Messori and reviewed by Lyndon DeVantier and three anonymous referees.
Abraham, J., Cheng, L., Mann, M. E., Trenberth, K., and von Schuckmann, K.: The ocean response to climate change guides both adaptation and mitigation efforts, Atmospheric and Oceanic Science Letters, 15, 100221, https://doi.org/10.1016/j.aosl.2022.100221, 2022.
Abrams, J. F., Huntingford, C., Williamson, M. S., Armstrong McKay, D. I., Boulton, C. A., Buxton, J. E., Sakschewski, B., Loriani, S., Zimm, C., Winkelmann, R., and Lenton, T. M.: Committed global warming risks triggering multiple climate tipping points, Earths Future, 11, e2022EF003250, https://doi.org/10.1029/2022EF003250, 2023.
Adelson, A. E., Altieri, A. H., Boza, X., Collin, R., Davis, K. A., Gaul, A., Giddings, S. N., Reed, V., and Pawlak, G.: Seasonal hypoxia and temperature inversions in a tropical bay, Limnol. Oceanogr., 67, 2174–2189, 2022.
Agostini, S., Houlbrèque, F., Biscéré, T., Harvey, B. P., Heitzman, J. M., Takimoto, R., Yamazaki, W., Milazzo, M., and Rodolfo-Metalpa, R.: Greater Mitochondrial Energy Production Provides Resistance to Ocean Acidification in “Winning” Hermatypic Corals, Front. Mar. Sci., 7, 600836, https://doi.org/10.3389/fmars.2020.600836, 2021.
Alderdice, R., Suggett, D. J., Cárdenas, A., Hughes, D. J., Kühl, M., Pernice, M., and Voolstra, C. R.: Divergent expression of hypoxia response systems under deoxygenation in reef-forming corals aligns with bleaching susceptibility, Glob. Change Biol., 27, 312–326, 2021.
Alderdice, R., Perna, G., Cárdenas, A., Hume, B. C., Wolf, M., Kühl, M., Pernice, M., Suggett, D. J., and Voolstra, C. R.: Deoxygenation lowers the thermal threshold of coral bleaching, Sci. Rep.-UK, 12, 18273, https://doi.org/10.1038/s41598-022-22604-3, 2022.
Altieri, A. H. and Gedan, K. B.: Climate change and dead zones, Glob. Change Biol., 21, 1395–1406, 2015.
Altieri, A. H., Harrison, S. B., Seemann, J., Collin, R., Diaz, R. J., and Knowlton, N.: Tropical dead zones and mass mortalities on coral reefs, P. Natl. Acad. Sci. USA, 114, 3660–3665, 2017.
Alvarez-Filip, L., Dulvy, N. K., Gill, J. A., Côté, I. M., and Watkinson, A. R.: Flattening of Caribbean coral reefs: region-wide declines in architectural complexity, P. Roy. Soc. B-Biol. Sci., 276, 3019–3025, https://doi.org/10.1098/rspb.2009.0339, 2009.
Alvarez-Filip, L., González-Barrios, F. J., Pérez-Cervantes, E., Molina-Hernández, A., and Estrada-Saldíva, N.: Stony coral tissue loss disease decimated Caribbean coral populations and reshaped reef functionality, Commun. Biol., 5, 440, https://doi.org/10.1038/s42003-022-03398-6, 2022.
Amir, H.: Status and trends of hard coral cover derived from long-term monitoring sites in the Maldives: 1998–2021, Maldives Marine Research Institute, Male', Republic of Maldives, http://www.mrc.gov.mv/assets/Uploads/MaldivesCoralReefReport-2022.pdf (last access: 22 January 2025), 2022.
Anthony, K. R. N.: Coral Reefs Under Climate Change and Ocean Acidification: Challenges and Opportunities for Management and Policy, Annu. Rev. Env. Resour., 41, 59–81, https://doi.org/10.1146/annurev-environ-110615-085610, 2016.
Anthony, K. R. N., Maynard, J. A., Diaz-Pulido, G., Mumby, P. J., Marshall, P. A., Cao, L., and Hoegh-Guldberg, O.: Ocean acidification and warming will lower coral reef resilience, Glob. Change Biol., 17, 1798–1808, https://doi.org/10.1111/j.1365-2486.2010.02364.x, 2011.
Anthony, K. R. N., Helmstedt, K. J., Bay, L. K., Fidelman, P., Hussey, K. E., Lundgren, P., Mead, D., McLeod, I. M., Mumby, P. J., Newlands, M., and Schaffelke, B.: Interventions to help coral reefs under global change – A complex decision challenge, PLoS ONE, 15, e0236399, https://doi.org/10.1371/journal.pone.0236399, 2020.
Armstrong-McKay, D. I., Staal, A., Abrams, J. F., Winkelmann, R., Sakschewski, B., Loriani, S., Fetzer, I., Cornell, S. E., Rockström, J., and Lenton, T. M.: Exceeding 1.5 °C global warming could trigger multiple climate tipping points, Science, 377, eabn7950, https://doi.org/10.1126/science.abn7950, 2022.
Aronson, R. B. and Precht, W. F.: Physical and biological drivers of coral reef dynamics, in: Coral Reefs at the Crossroads, vol. 6, edited by: Hubbard, D. K., Rogers, C. S., Lipps, J. H., and Stanley Jr., G. D., Springer, 261–275, ISBN: 978-94-017-7565-6, 2016.
Auger, M., Morrow, R., Kestenare, E., Sallée, J. B., and Cowley, R.: Southern Ocean in-situ temperature trends over 25 years emerge from interannual variability, Nat. Commun., 12, 514, https://doi.org/10.1038/s41467-020-20781-1, 2021.
Babcock, R. C., Dambacher, J. M., Morello, E. B., Plagányi, É. E., Hayes, K. R., Sweatman, H. P., and Pratchett, M. S.: Assessing different causes of crown-of-thorns starfish outbreaks and appropriate responses for management on the Great Barrier Reef, PloS one, 11, e0169048, https://doi.org/10.1371/journal.pone.0169048, 2016.
Baldock, T. E., Golshani, A., Callaghan, D. P., Saunders, M. I., and Mumby, P. J.: Impact of sea-level rise and coral mortality on the wave dynamics and wave forces on barrier reefs, Mar. Pollut. Bull., 83, 155–164, 2014.
Ban, S., Graham, N., and Connolly, S.: Evidence for multiple stressor interactions and effects on coral reefs, Glob. Change Biol., 20, 681–697, https://doi.org/10.1111/gcb.12453, 2013.
Bates, A. E., Cooke, R. S., Duncan, M. I., Edgar, G. J., Bruno, J. F., Benedetti-Cecchi, L., Côté, I. M., Lefcheck, J. S., Costello, M. J., Barrett, N., Bird, T. J., Fenberg, P. B., and Stuart-Smith, R. D.: Climate resilience in marine protected areas and the “Protection Paradox”, Biol. Conserv., 236, 305–314, https://doi.org/10.1016/j.biocon.2019.05.005, 2019.
Beyer, H. L., Kennedy, E. V., Beger, M., Chen, C. A., Cinner, J. E., Darling, E. S., Eakin, C. M., Gates, R. D., Heron, S. F., Knowlton, N., Obura, D., Palumbi, S. R., Possingham, H. P., Puotinen, M., Runting, R. K., Skirving, W. J., Spalding, M., Wilson, K. A. Wood, S., Veron, J. E., and Hoegh-Guldberg, O.: Risk-sensitive planning for conserving coral reefs under rapid climate change, Conserv. Lett., 11, e12587, https://doi.org/10.1111/conl.12587, 2018.
Bland, L. M., Rowland, J. A., Regan, T. J., Keith, D. A., Murray, N. J., Lester, R. E., Linn, M., Rodriguez, J. P., and Nicholson, E.: Developing a standardized definition of ecosystem collapse for risk assessment, Front. Ecol. Environ., 16, 29–36, https://doi.org/10.1002/fee.1747, 2018.
Bongaerts, P. and Smith, T.: Beyond the “Deep Reef Refuge” Hypothesis: A Conceptual Framework to Characterize Persistence at Depth, in: Mesophotic Coral Ecosystems, vol. 12, edited by: Loya, Y., Puglise, K. A., and Bridge, T. C. L., Springer, Cham., ISBN: 978-3-319-92734-3, https://doi.org/10.1007/978-3-319-92735-0_45, 2019.
Briggs, N. D., Page, C. A., Giuliano, C., Alessi, C., Hoogenboom, M., Bay, L. K., and Randall, C. J.: Dissecting coral recovery: bleaching reduces reproductive output in Acropora millepora, Coral Reefs, 43, 557–569, 2024.
Brodie, J. E., Kroon, F. J., Schaffelke, B., Wolanski, E. C., Lewis, S. E., Devlin, M. J., Bohnet, I. C., Bainbridge, Z. T., Waterhouse, J., and Davis, A. M.: Terrestrial pollutant runoff to the Great Barrier Reef: an update of issues, priorities and management responses, Mar. Pollut. Bull., 65, 81–100, 2012.
Brown, B. E., Dunne, R. P., Somerfield, P. J., Edwards, A. J., Simons, W. J. F., Phongsuwan, N., Putchim, L., Anderson, L., and Naeije, M. C.: Long-term impacts of rising sea temperature and sea level on shallow water coral communities over a ∼40 year period, Sci. Rep.-UK, 9, 8826, https://doi.org/10.1038/s41598-019-45188-x, 2019.
Bruno, J., Selig, E., Casey, K., Page, C., Willis, B., Harvell, C., Sweatman, H., and Melendy, A.: Thermal stress and coral cover as drivers of coral disease outbreaks, PLoS Biol., 5, e124, https://doi.org/10.1371/journal.pbio.0050124, 2007.
Bruno, J. F., Sweatman, H., Precht, W. F., Selig, E. R., and Schutte, V. G. W.: Assessing evidence of phase shifts from coral to macroalgal dominance on coral reefs, Ecology, 90, 1478–1484, 2009.
Bruno, J. F., Côté, I. M., and Toth, L. T.: Climate change, coral loss, and the curious case of the parrotfish paradigm: why don't marine protected areas improve reef resilience?, Annu. Rev. Mar. Sci., 11, 307–334, https://doi.org/10.1146/annurev-marine-010318-095300, 2019.
Burke, S., Pottier, P., Lagisz, M., Macartney, E. L., Ainsworth, T., Drobniak, S. M., and Nakagawa, S.: The impact of rising temperatures on the prevalence of coral diseases and its predictability: A global meta-analysis, Ecol. Lett., 26, 1466–1481, https://doi.org/10.1111/ele.14266, 2023.
Caldeira, K. and Wickett, M. E.: Anthropogenic carbon and ocean pH, Nature, 425, 365–365, 2003.
Carballo, J. L., Bautista, E., Nava, H., Cruz-Barraza, J. A., and Chávez, J. A.: Boring sponges, an increasing threat for coral reefs affected by bleaching events, Ecol. Evol., 3, 872–886, https://doi.org/10.1002/ece3.452, 2013.
Carrigan, A. D. and Puotinen, M.: Tropical cyclone cooling combats region-wide coral bleaching, Glob. Change Biol., 20, 1604–1613, 2014.
Cavada-Blanco, F., Croquer, A., Vermeij, M., Goergen, L., and Rodriguez, R.: Dendrogyra cylindrus. The IUCN Red List of Threatened Species 2022: e.T133124A129721366, https://doi.org/10.2305/IUCN.UK.2022-2.RLTS.T133124A129721366.en, 2022.
Cesar, H., Burke, L., and Pet-Soede, L.: The economics of worldwide coral reef degradation, International Coral Reef Action Network, https://www.icran.org/wp-content/uploads/2022/11/cesardegradationreport.pdf (last access: 22 January 2025), 2003.
Cheal, A. J., MacNeil, M. A., Emslie, M. J., and Sweatman, H.: The threat to coral reefs from more intense cyclones under climate change, Glob. Change Biol., 23, 1511–1524, https://doi.org/10.1111/gcb.13593, 2017.
Cheng, L., von Schuckmann, K., Abraham, J. P., Trenberth, K. E., Mann, M. E., Zanna, L., England, M. H., Zika, J. D., Fasullo, J. T., Yu, Y., Pan, Y., Zhu, J., Newsom, E. R., Bronselaer, B., and Lin, X.: Past and future ocean warming, Nat. Rev. Earth Environ., 3, 776–794, 2022.
Cinner, J. E., McClanahan, T. R., MacNeil, M. A., Graham, N. A., Daw, T. M., Mukminin, A., Feary, D. A., Rabearisoa, A. L., Wamukota, A., Jiddawi, N., Campbell, S. J., Baird, A. H., Januchowski-Hartley, F. A., Hamed, S., Lahari, R., Morove, T., and Kuange, J.: Comanagement of coral reef social-ecological systems, P. Natl. Acad. Sci. USA, 109, 5219–5222, https://doi.org/10.1073/pnas.1121215109, 2012.
Cinner, J. E., Huchery, C., Hicks, C. C., Daw, T. M., Marshall, N., Wamukota, A., and Allison, E. H.: Changes in adaptive capacity of Kenyan fishing communities, Nat. Clim. Change, 5, 872–876, https://doi.org/10.1038/nclimate2690, 2015.
Ciracì, E., Rignot, E., Scheuchl, B., Tolpekin, V., Wollersheim, M., An, L., Milillo, P., Bueso-Bello, J., Rizzoli, P., and Dini, L.: Melt rates in the kilometer-size grounding zone of Petermann Glacier, Greenland, before and during a retreat, P. Natl. Acad. Sci. USA, 120, e2220924120, https://doi.org/10.1073/pnas.2220924120, 2023.
Claar, D. C., Szostek, L., McDevitt-Irwin, J. M., Schanze, J. J., and Baum, J. K.: Global patterns and impacts of El Niño events on coral reefs: A metaanalysis, PLoS, 13, 1–22, https://doi.org/10.1371/journal.pone.0190957, 2018.
Comeau, S., Edmunds, P. J., Spindel, N. B., and Carpenter, R. C.: Fast coral reef calcifiers are more sensitive to ocean acidification in short-term laboratory incubations, Limnol. Oceanogr., 59, 1081–1091, 2014.
Comeau, S., Cornwall, C. E., DeCarlo, T. M., Krieger, E., and McCulloch, M. T.: Similar controls on calcification under ocean acidification across unrelated coral reef taxa, Glob. Change Biol., 24, 4857–4868, 2018.
Comeau, S., Cornwall, C. E., DeCarlo, T. M., Doo, S. S., Carpenter, R. C., and McCulloch, M. T.: Resistance to ocean acidification in coral reef taxa is not gained by acclimatization, Nat. Clim. Change, 9, 477–483, 2019.
Comeau, S., Cornwall, C. E., Shlesinger, T., Hoogenboom, M., Mana, R., McCulloch, M. T., and Rodolfo-Metalpa, R.: pH variability at volcanic CO2 seeps regulates coral calcifying fluid chemistry, Glob. Change Biol., 28, 2751–2763, 2022.
Cornwall, C. E., Comeau, S., Kornder, N. A., Perry, C. T., van Hooidonk, R., DeCarlo, T. M., Pratchett, M. S., Anderson, K. D., Browne, N., Carpenter, R., Diaz-Pulido, G., D'Olivo, J. P., Doo, S. S., Figueiredo, J., Fortunato, S. A. V., Kennedy, E., Lantz, C. A., McCulloch, M. T., González-Rivero, M., Schoepf, V., Smithers, S. G., and Lowe, R. J.: Global declines in coral reef calcium carbonate production under ocean acidification and warming, P. Natl. Acad. Sci. USA, 118, e2015265118, https://doi.org/10.1073/pnas.2015265118, 2021.
Cornwall, C. E., Comeau, S., Donner, S. D., Perry, C., Dunne, J., van Hooidonk, R., Ryan, J. S., and Logan, C. A.: Coral adaptive capacity insufficient to halt global transition of coral reefs into net erosion under climate change, Glob. Change Biol., 29, 3010–3018, 2023.
Cornwall, C. E., Comeau, S., and Harvey, B. P.: Are physiological and ecosystem-level tipping points caused by ocean acidification? A critical evaluation, Earth Syst. Dynam., 15, 671–687, https://doi.org/10.5194/esd-15-671-2024, 2024.
Cramer, K., Jackson, J., Donovan, M., Greenstein B., Korpanty, C., Cook, J., and Pandolfi, J.: Widespread loss of Caribbean acroporid corals was underway before coral bleaching and disease outbreaks, Sci. Adv., 6, eaax9395, https://doi.org/10.1126/sciadv.aax9395, 2020.
Darling, E. S., McClanahan, T. R., and Côté, I. M.: Combined effects of two stressors on Kenyan coral reefs are additive or antagonistic, not synergistic, Conserv. Lett., 3, 122–130, https://doi.org/10.1111/j.1755-263X.2009.00089.x, 2010.
Darling, E. S., McClanahan, T. R., Maina, J., Gurney, G. G., Graham, N. A. J., Januchowski-Hartley, F., Cinner, J. E., Mora, C., Hicks, C. C., Maire, E., Puotinen, M., Skirving, W. J., Adjeroud, M., Ahmadia, G., Arthur, R., Bauman, A. G., Beger, M., Berumen, M. L., Bigot, L., Bouwmeester, J., Brenier, A., Bridge, T. C. L., Brown, E., Campbell, S. J., Cannon, S., Cauvin, B., Chen, C. A., Claudet, J., Denis, V., Donner, S., Estradivari, Fadli, N., Feary, D. A., Fenner, D., Fox, H., Franklin, E. C., Friedlander, A., Gilmour, J., Goiran, C., Guest, J., Hobbs, J. A., Hoey, A. S., Houk, P., Johnson, S., Jupiter, S. D., Kayal, M., Kuo, C., Lamb, J., Lee, M. A. C., Low, J., Muthiga, N., Muttaqin, E., Nand, Y., Nash, K. L., Nedlic, O., Pandolfi, J. M., Pardede, S., Patankar, V., Penin, L., Ribas-Deulofeu, L., Richards, Z., Roberts, T. E., Rodgers, K. S., Saufuan, C. D. M., Sala, E., Shedrawi, G., Sin, T. M., Smallhorn-West, P., Smith, J. E., Sommer, B., Steinberg, P. D., Sutthacheep, M., Tan, C. H. J., Williams, G. J., Willson, S., Yeemin, T., Bruno, J. F., Fortin, M., Krkosek, M., and Mouillot, D.: Social–environmental drivers inform strategic management of coral reefs in the Anthropocene, Nat. Ecol. Evol., 3, 1341–1350, https://doi.org/10.1038/s41559-019-0953-8, 2019.
Davis, K. L., Colefax, A. P., Tucker, J. P. Kelaher B. P., and Santos, I. R.: Global coral reef ecosystems exhibit declining calcification and increasing primary productivity, Commun. Earth Environ., 2, 105, https://doi.org/10.1038/s43247-021-00168-w, 2021.
De'ath, G. and Fabricius, K.: Water quality as a regional driver of coral biodiversity and macroalgae on the Great Barrier Reef, Ecol. Appl., 20, 840–850, https://doi.org/10.1890/08-2023.1, 2010.
Deutloff, J. E., Held, H., and Lenton, T. M.: The risky middle of the road – probabilities of triggering climate tipping points and how they increase due to tipping points within the Earth's carbon cycle, EGUsphere [preprint], https://doi.org/10.5194/egusphere-2023-1469, 2023.
Diaz, R. J. and Rosenberg, R.: Spreading dead zones and consequences for marine ecosystems, Science, 321, 926–929, 2008.
Dinsdale, E. A. and Rohwer, F.: Fish or germs? Microbial dynamics associated with changing trophic structures on coral reefs. Coral reefs: an ecosystem in transition, Springer Netherlands, Dordrecht, 231–240, https://doi.org/10.1007/978-94-007-0114-4_16, 2011
Dixon, A. M., Forster, P. M., and Beger, M.: Coral conservation requires ecological climate-change vulnerability assessments, Front. Ecol. Environ., 19, 243–250, https://doi.org/10.1002/fee.2312, 2021.
Dixon, A. M., Puotinen, M., Ramsay, H. A., and Beger, M.: Coral reef exposure to damaging tropical cyclone waves in a warming climate, Earths Future, 10, e2021EF002600, https://doi.org/10.1029/2021EF002600, 2022.
Donovan, M. K., Burkepile, D. E., Kratochwill, C., Shlesinger, T., Sully, S., Oliver, T. A., Hodgson, G., Freiwald, J., and van Woesil, R.: Local conditions magnify coral loss after marine heatwaves, Science, 372, 977–980, https://doi.org/10.1126/science.abd9464, 2021.
Eakin, C. M., Devotta, D., Heron, S. F., Connolly, S., Liu, G., Geiger, E., Couch, C., Donner, S., Gilmour, J., Gonzalez-Rivero, M., Gudka, M., Harrison, H., Hodgson, G., Hoegh-Guldberg, O., Hoey, A. S., Hoogenboom, M., Hughes, T. P., Johnson, M., Kerry, J., Mihaly, J., Muñiz-Castillo, A., Obura, D., Pratchett, M., Rivera-Sosa, A., Ross, C., Stein, J., Thompson, A., Torda, G., Viehman, T. S., Walter, C., Wilson, S. K., Marsh, B., Spady, B., Dyer, N., Adam, T., Alidoostsalimi, M., Alidoostsalimi, P., Alvarez-Filip, L., Álvarez-Noriega, M., Bahr, K., Barnes, P., Sandoval, J. B., Baum, J., Bauman, A., Beger, M., Berry, K., Bessell-Browne, P., Bigot, L., Bonito, V., Brodnicke, O., Burdick, D., Burkepile, D., Burt, A., Burt, J. A., Butler, I., Caldwell, J., Chancerelle, Y., Chen, C., Allen Cherh, K. L., Childress, M., Coken, D., Coward, G., Crabbe, M. J., Dallison, T., Dalton, S., De Carlo, T. M., Drury, C., Drysdale, I., Edwards, C., Eggertsen, L., Elma, E., Ennis, R., Evans, R., Eyal, G., Fenner, D., Figueroa-Zavala, B., Fisch, J., Fox, M. D., Gadoutsis, E., Gilbert, A., Halford, A., Heintz, T., Hewlett, J., Hobbs, J. P. A., Hoot, W., Houk, P., Johnston, L., Johnston, M., Kayanne, H., Kennedy, E., Kikuchi, R. K. P., Kloiber, Ulrike Koike, H., Kramer, L., Kuo, C. Y., Lang, J., Leadbeater, A., Leão, Z., Lee, J., Lewis, C., Lirman, D., Longo, G., MacDonald, C., Mangubhai, S., da Silva, I., Mason-Parker, C., McDonough, V., McField, M., Mello, T., Agathe, C. M., Moldzio, S., Monroe, A., Montefalcone, M., Moses, K., Mostafavi, P. G., Moura, R., Munasinghe, C., Nakamura, T., Nicet, J. B., Nuttall, M., Oliveira, M., Oxenford, H., Pandolfi, J. M., Patankar, V., Perez, D., Perera, N., Prabuning, D., Precht, W., Raj, K. D., Reimer, J. D., Richardson, L., Rotjan, R., Ryan, N., Salm, R., Sandin, S. A., Schopmeyer, S., Shokri, M., Smith, J., Smith, K., Smith, S. R., Smith, T., Sommer, B., Soto, M., Sykes, H., Tagarino, K., Teoh, M., Thai, M., Toh, T., Tredinnick, A., Tso, A., Tyley, H., Ussi, A., Vaterlaus, C., Vermeij, M., Vo, S. T., Voolstra, C. R., Wee, H. B., Weiler, B., Yahya, S., Yeemin, T., Ziegler, M., Kimura, T., and Manzello, D.: The 2014–17 Global Coral Bleaching Event: The Most Severe and Widespread Coral Reef Destruction, Research Square, https://doi.org/10.21203/rs.3.rs-1555992/v1, 2022.
Eddy, T. D., Lam, V. W. Y., Reygondeau, G., Cisneros-Montemayor, A. M., Greer, K., Palomares, M. L. D., Bruno, J. F., Ota, Y., and Cheung, W. W. L.: Global decline in capacity of coral reefs to provide ecosystem services, One Earth, 4, 1278–1285, https://doi.org/10.1016/j.oneear.2021.08.016, 2021.
England, M. R., Polvani, L. M., Sun, L., and Deser, C.: Tropical climate responses to projected Arctic and Antarctic sea-ice loss, Nat. Geosci., 13, 275–281, https://doi.org/10.1038/s41561-020-0546-9, 2020.
Estrada-Saldívar, N., Quiroga-García, B. A., Pérez-Cervantes, E., Rivera-Garibay, O. O., and Alvarez-Filip, L.: Effects of the Stony Coral Tissue Loss Disease outbreak on coral communities and the benthic composition of Cozumel reefs, Front. Mar. Sci., 8, 632777, https://doi.org/10.3389/fmars.2021.632777, 2021.
Fabricius, K. E.: Effects of terrestrial runoff on the ecology of corals and coral reefs: review and synthesis, Mar. Pollut. Bull., 50, 125–146, https://doi.org/10.1016/j.marpolbul.2004.11.028, 2005.
Fabricius, K. E., Langdon, C., Uthicke, S., Humphrey, C., Noonan, S., De'ath, G., Okazaki, R., Muehllehner, N., Glas, M. S., and Lough. J. M.: Losers and winners in coral reefs acclimatized to elevated carbon dioxide concentrations, Nat. Clim. Change, 1, 165–169, 2011.
Feely, R. A., Sabine, C. L., Lee, K., Berelson, W., Kleypas, J., Fabry, V. J., and Millero, F. J.: Impact of anthropogenic CO2 on the CaCO3 system in the oceans, Science, 305, 362–366, 2004.
Fisher, R., Bessell-Browne, P., and Jones, R.: Synergistic and antagonistic impacts of suspended sediments and thermal stress on corals, Nat. Commun., 10, 2346, https://doi.org/10.1038/s41467-019-10288-9, 2019.
Fletcher, C., Ripple, W. J., Newsome, T., Barnard, P., Beamer, K., Behl, A., Bowen, J., Cooney, M., Crist, E., Field, C., Hiser, K., Karl, D. M., King, D. A., Mann, M. E., McGregor, D. P., Mora, C., Oreskes, N., and Wilson, M.: Earth at risk: An urgent call to end the age of destruction and forge a just and sustainable future, PNAS Nexus, 3, 106, 2024.
Fox, M. D., Cohen, A. L., Rotjan, R. D., Mangubhai, S., Sandin, S. A., Smith, J. E., Thorrold, S. R., Dissly, L., Mollica, N. R., and Obura, D.: Increasing coral reef resilience through successive marine heatwaves, Geophys. Res. Lett., 48, e2021GL094128, https://doi.org/10.1029/2021GL094128, 2021.
Frieler, K., Meinshausen, M., Golly, A., Mengel, M., Lebek, K., Donner, S. D., and Hoegh-Guldberg, O.: Limiting global warming to 2 °C is unlikely to save most coral reefs, Nat. Clim. Change, 3, 165–170, https://doi.org/10.1038/nclimate1674, 2013.
Gardner, T. A., Côté, I. M., Gill, J. A., Grant, A., and Watkinson, A. R.: Hurricanes and Caribbean coral reefs: impacts, recovery patterns, and role in long-term decline, Ecology, 86, 174–184, https://doi.org/10.1890/04-0141, 2005.
Genin, A., Levy, L., Sharon, G., and Diamant, A.: Rapid onsets of warming events trigger mass mortality of coral reef fish, P. Natl. Acad. Sci. USA, 117, 25378–25385, https://doi.org/10.1073/pnas.2009748117, 2020.
Good, A. M. and Bahr, K. D.: The coral conservation crisis: interacting local and global stressors reduce reef resiliency and create challenges for conservation solutions, SN Appl. Sci., 3, 312, https://doi.org/10.1007/s42452-021-04319-8, 2021.
Goreau, T. J. and Hayes, R. L.: 2023 Record marine heat waves: coral reef bleaching HotSpot maps reveal global sea surface temperature extremes, coral mortality, and ocean circulation changes, Oxf. Open Clim. Chang., 4, kgae005, https://doi.org/10.1093/oxfclm/kgae005, 2024.
Grigg, R.: The Darwin Point: a conceptual and historical review, Environmental Science, http://www.pelagicos.net/BIOL3010/readings/Grigg_2008.pdf (last access: 22 January 2025), 2008.
Guannel, G., Arkema, K., Ruggiero, P., and Verutes, G.: The Power of Three: Coral Reefs, Seagrasses and Mangroves Protect Coastal Regions and Increase Their Resilience, PLoS One, 11, e0158094, https://doi.org/10.1371/journal.pone.0158094, 2016.
Hansen, J., Nazarenko, L., Ruedy, R., Sato, M., Willis, J., Del Genio, A., Koch, D., Lacis, A., Lo, K., Menon, S., Novakov, T., Perlwitz, J., Russell, G., Schmidt, G. A., and Tausnev, N.: Earth's energy imbalance: Confirmation and implications, Science, 308, 1431–1435, 2005.
Heinze, C., Blenckner, T., Martins, H., and Wilson, S.: The quiet crossing of ocean tipping points, P. Natl. Acad. Sci. USA, 118, e2008478118, https://doi.org/10.1073/pnas.2008478118, 2021.
Herbert-Read, J. E., Thornton, A., Amon, D. J., Birchenough, S. N., Côté, I. M., Dias, M. P., Godley, B. J., Keith, S. A., McKinley, E., Peck, L. S., Calado, R., Defeo, O., Degraer, S., Johnston, E. L., Kaartokallio, H., Macreadie, P. I., Metaxas, A., Muthumbi, A. W. N., Obura, D. O., Paterson, D. M., Piola, A. R., Richardson, A. J., Schloss, I. R., Snelgrove, P. V. R., Stewart, B. D., Thompson, P. M., Watson, G. J., Worthington, T. A., Yasuhara, M., and Sutherland, W. J.: A global horizon scan of issues impacting marine and coastal biodiversity conservation, Nat. Ecol. Evol., 6, 1262–1270, 2022.
Hoegh-Guldberg, O., Poloczanska, E. S., Skirving, W., and Dove, S.: Coral Reef Ecosystems Under Climate Change and Ocean Acidification, Front. Mar. Sci., 4, 158, https://doi.org/10.3389/fmars.2017.00158, 2017.
Hoegh-Guldberg, O., Jacob, D., Taylor, M., Bindi, M., Brown, S., Camilloni, I., Diedhiou, A., Djalante, R., Ebi, K. L., Engelbrecht, F., Guiot, J., Hijioka, Y., Mehrotra, S., Payne, A., Seneviratne, S. I., Thomas, A., Warren, R., and Zhou, G.: Impacts of 1.5 °C Global Warming on Natural and Human Systems, in: Global Warming of 1.5 °C. An IPCC Special Report on the impacts of global warming of 1.5 °C above pre-industrial levels and related global greenhouse gas emission pathways, in the context of strengthening the global response to the threat of climate change, sustainable development, and efforts to eradicate poverty, edited by: Masson-Delmotte, V., Zhai, P., Pörtner, H.-O., Roberts, D., Skea, J., Shukla, P. R., Pirani, A., Moufouma-Okia, W., Péan, C., Pidcock, R., Connors, S., Matthews, J. B. R., Chen, Y., Zhou, X., Gomis, M. I., Lonnoy, E., Maycock, T., Tignor, M., and Waterfield, T., Cambridge University Press, Cambridge, UK and New York, NY, USA, 175–312, https://doi.org/10.1017/9781009157940.005, 2018.
Hooijer, A. and Vernimmen, R.: Global LiDAR land elevation data reveal greatest sea-level rise vulnerability in the tropics, Nat. Commun., 12, 3592, https://doi.org/10.1038/s41467-021-23810-9, 2021.
Houk, P., Yalon, A., Maxin, S., Starsinic, C., McInnis, A., Gouezo, M., Golbuu, Y., and van Woesik, R.: Predicting coral-reef futures from El Niño and Pacific Decadal Oscillation events, Sci. Rep.-UK, 10, 7735, https://doi.org/10.1038/s41598-020-64411-8, 2020.
Howard, R. D., Schul, M. D., Rodriguez Bravo, L. M., Altieri, A. H., and Meyer, J. L.: Shifts in the coral microbiome in response to in situ experimental deoxygenation, App. Environ. Microbiol., 89, e00577-23, https://doi.org/10.1128/aem.00577-23, 2023.
Howe-Kerr, L. I., Grupstra, C. G. B., Rabbitt, K. M. Conetta, D., Coy, S. R., Klinges, J. G., Maher, R. L., McConnell, K. M., Meiling, S. S., Messyasz, A., Schmeltzer, E. R., Seabrook, S., Sims, J. A., Veglia, A. J., Thurber, A. R., Vega Thurber R. L., and Correa, A. M. S.: Viruses of a key coral symbiont exhibit temperature-driven productivity across a reefscape, ISME Commun., 3, 27, https://doi.org/10.1038/s43705-023-00227-7, 2023.
Hughes, T. P., Kerry, J. T., Álvarez-Noriega, M., Álvarez-Romero, J. G., Anderson, K. D., Baird, A. H., Babcock, R. C., Beger, M., Bellwood, D. R., Berkelmans, R., Bridge, T. C., Butler, I. R., Byrne, M., Cantin, N. E., Comeau, S., Connolly, S. R., Cumming, G. S., Dalton, S. J. Diaz-Pulido, G., Eakin, C. M., Figueira, W. F., Gilmour, J. P., Harrison, H. B., Heron, S. F., Hoey, A. S., Hobbs, J.-P. A., Hoogenboom, M. O., Kennedy, E. V., Kuo, C., Lough, J. M., Lowe, R. J., Liu, G., McCulloch, M. T., Malcolm, H. A., McWilliam, M. J., Pandolfi, J. M., Pears, R. J., Pratchett, M. S., Schoepf, V., Simpson, T., Skirving, W. J., Sommer, B., Torda, G. Wachenfeld, D. R., Willis, B. L., and Wilson, S. K.: Global warming and recurrent mass bleaching of corals, Nature, 543, 373–377, https://doi.org/10.1038/nature21707, 2017.
Hughes, T. P., Anderson, K. D., Connolly, S. R., Heron, S. F., Kerry, J. T., Lough, J. M., Baird, A. H., Baum, J. K., Berumen, M. L., Bridge, T. C., Claar, D. C., Eakin, C. M., Gilmour, J. P., Graham, N. A. J., Harrison, H., Hobbs, J.-P. A., Hoey, A. S., Hoogenboom, M., Lowe, R. J., McCulloch, M. T., Pandolfi, J. M., Pratchett, M., Schoepf, V., Torda, G., and Wilson, S. K.: Spatial and temporal patterns of mass bleaching of corals in the Anthropocene, Science, 359, 80–83, https://doi.org/10.1126/science.aan8048, 2018a.
Hughes, T. P., Kerry, J. T., Baird, A. H., Connolly, S. R., Dietzel, A., Eakin, C. M., Heron, S. F., Hoey, A. S., Hoogenboom, M. O., Liu, G., McWilliam, M. J., Pears, R. J., Pratchett, M. S., Skirving, W. J., Stella, J. S., and Torda, G.: Global warming transforms coral reef assemblages, Nature, 556, 492–496, 2018b.
Hughes, D. J., Alderdice, R., Cooney, C., Kühl, M., Pernice, M., Voolstra, C. R., and Suggett, D. J.: Coral reef survival under accelerating ocean deoxygenation, Nat. Clim. Change, 10, 296–307, 2020.
Hughes, T. P., Baird, A. H., Morrison, T. H., and Torda, G.: Principles for coral reef restoration in the anthropocene, One Earth, 6, 656–665, 2023.
IPBES: Global assessment report on biodiversity and ecosystem services of the Intergovernmental Science-Policy Platform on Biodiversity and Ecosystem Services, edited by: Brondizio, E. S., Settele, J., Díaz, S., and Ngo, H. T., IPBES secretariat, Bonn, Germany, 1148 pp., https://doi.org/10.5281/zenodo.3831673, 2019.
IPCC: Global Warming of 1.5 °C. An IPCC Special Report on the impacts of global warming of 1.5 °C above pre-industrial levels and related global greenhouse gas emission pathways, in the context of strengthening the global response to the threat of climate change, sustainable development, and efforts to eradicate poverty, edited by: Masson-Delmotte, V., Zhai, P., Pörtner, H.-O., Roberts, D., Skea, J., Shukla, P. R., Pirani, A., Moufouma-Okia, W., Péan, C., Pidcock, R., Connors, S., Matthews, J. B. R., Chen, Y., Zhou, X., Gomis, M. I., Lonnoy, E., Maycock, T., Tignor, M., and Waterfield, T. Cambridge University Press, Cambridge, UK and New York, NY, USA, 616 pp., https://doi.org/10.1017/9781009157940, 2018.
IPCC: Climate Change 2021: The Physical Science Basis. Contribution of Working Group I to the Sixth Assessment Report of the Intergovernmental Panel on Climate Change, edited by: Masson-Delmotte, V., Zhai, P., Pirani, A., Connors, S. L., Pen, C., Berger, S., Caud, N., Chen, Y. Goldfarb, L., Gomis, M. I., Huang, M., Leitzell, K., Lonnoy, E., Matthews, J. B. R., Maycock, T. K., Waterfield, T., Yelekçi, O., Yu, R., and Zhou, B., Cambridge University Press, Cambridge, United Kingdom and New York, NY, USA, 2391 pp., https://doi.org/10.1017/9781009157896, 2021.
IPCC: Climate Change 2022: Impacts, Adaptation and Vulnerability. Contribution of Working Group II to the Sixth Assessment Report of the Intergovernmental Panel on Climate Change, edited by: Pörtner, H.-O., Roberts, D. C., Tignor, M., Poloczanska, E. S., Mintenbeck, K., Alegría, A., Craig, M., Langsdorf, S., Löschke, S., Möller, V., Okem, A., and Rama, B., Cambridge University Press, Cambridge, UK and New York, NY, USA, 3056 pp., https://doi.org/10.1017/9781009325844, 2022.
Johnson, J. V., Dick, J. T. A., Pincheira-Donoso, D., and Bates, A. E.: Local anthropogenic stress does not exacerbate coral bleaching under global climate change, Glob. Ecol. Biogeogr., , 31, 1228–1236, https://doi.org/10.1111/geb.13506, 2022.
Johnson, M. D., Scott, J. J., Leray, M., Lucey, N., Bravo, L. M. R., Wied, W. L., and Altieri, A. H.: Rapid ecosystem-scale consequences of acute deoxygenation on a Caribbean coral reef, Nat. Commun., 12, 4522, https://doi.org/10.1038/s41467-021-24777-3, 2021a.
Johnson, M. D., Swaminathan, S. D., Nixon, E. N., Paul, V. J., and Altieri, A. H.: Differential susceptibility of reef-building corals to deoxygenation reveals remarkable hypoxia tolerance, Sci. Rep.-UK, 11, 23168, https://doi.org/10.1038/s41598-021-01078-9, 2021b.
Kalmus, P., Ekanayaka, A., Kang, E., Baird, M., and Gierach, M.: Past the precipice? Projected coral habitability under global heating, Earths Future, 10, e2021EF002608, https://doi.org/10.1029/2021EF002608, 2022.
Ke, P., Ciais, P., Sitch, S., Li, W., Bastos, A., Liu, Z., Xu, Y., Gui, X., Bian, J., Goll, D. S., Xi, Y., Li, W., O'Sullivan, M., Goncalves de Souza, J., Friedlingstein, P., and Chevallier, F.: Low latency carbon budget analysis reveals a large decline of the land carbon sink in 2023, Nat. Sci. Rev., 11, nwae367, https://doi.org/10.1093/nsr/nwae367, 2024.
Klein, S. G., Roch, C., and Duarte, C. M.: Systematic review of the uncertainty of coral reef futures under climate change, Nat. Commun., 15, 2224, https://doi.org/10.1038/s41467-024-46255-2, 2024.
Kleypas, J., Allemand, D., Anthony, K., Baker, A. C., Beck, M. W., Hale, L. Z., Hilmi, N., Hoegh-Guldberg, O., Hughes, T., Kaufman, L., Kayanne, H., Magnan, A. K., Mcleod, E., Mumby, P., Palumbi, S., Richmond, R. H., Rinkevich, B., Steneck, R. S., Voolstra, C. R., Wachenfeld, D., and Gattuso, J. P.: Designing a blueprint for coral reef survival, Biol. Conserv., 257, 109107, https://doi.org/10.1016/j.biocon.2021.109107, 2021.
Knowlton, N., Grottoli, A. G., Kleypas, J., Obura, D., Corcoran, E., de Goeij, J. M., Felis, T., Harding, S., Mayfield, A., Miller, M., Osuka, K., Peixoto, R., Randall, C. J., Voolstra, C. R., Wells, S., Wild, C., and Ferse, S.: Rebuilding Coral Reefs: A Decadal Grand Challenge, International Coral Reef Society and Future Earth Coasts, 56 pp., https://doi.org/10.53642/NRKY9386, 2021.
Kornder, N. A., Riegl, B. M., and Figueiredo, J.: Thresholds and drivers of coral calcification responses to climate change, Glob. Change Biol., 24, 5084–5095, https://doi.org/10.1111/gcb.14431, 2018.
Kroon, F. J., Schaffelke, B., and Bartley, R.: Informing policy to protect coastal coral reefs: Insight from a global review of reducing agricultural pollution to coastal ecosystems, Mar. Pollut. Bull., 85, 33–41, 2014.
Laffoley, D. and Baxter, J. M.: Explaining ocean warming: causes, scale, effects and consequences. 3.8 Impacts and effects of ocean warming on coral reefs, IUCN, https://doi.org/10.2305/IUCN.CH.2016.08.en, 2016.
Laffoley, D. and Baxter, J. M.: Ocean deoxygenation: everyone's problem – causes, impacts, consequences and solutions, IUCN, https://doi.org/10.2305/IUCN.CH.2019.13.en, 2019.
Laffoley, D., Baxter, J. M., Amon, D. J., Claudet, J., Downs, C. A., Earle, S. A., Gjerde, K. M., Hall-Spencer, J. M., Koldewey, H. J., Levin, L. A., Reid, C. P., Roberts, C. M., Sumaila, R. U., Taylor, M. L., Thiele, T., and Woodall, L. C.: The forgotten ocean: Why COP26 must call for vastly greater ambition and urgency to address ocean change, Aquat. Conserv., 32, 217–228, 2022.
Lamb, J. B., Willis, B. L., Fiorenza, E. A., Couch, C. S., Howard, R., Rader, D. N., True, J. D., Kelly, L. A., Ahmad, A., Jompa, J., and Harvell, C. D.: Plastic waste associated with disease on coral reefs, Science, 359, 460–462, https://doi.org/10.1126/science.aar3320, 2018.
Le Nohaïc, M., Ross, C. L., Cornwall, C. E., Comeau, S., Lowe, R., McCulloch, M. T., and Schoepf, V.: Marine heatwave causes unprecedented regional mass bleaching of thermally resistant corals in northwestern Australia, Sci. Rep.-UK, 7, 14999, https://doi.org/10.1038/s41598-017-14794-y, 2017.
Lenton, T. M., Armstrong McKay, D. I., Loriani, S., Abrams, J. F., Lade, S. J., Donges, J. F., Milkoreit, M., Powell, T., Smith, S. R., Zimm, C., Buxton, J. E., Bailey, E., Laybourn, L., Ghadiali, A., and Dyke, J. G. (Eds.): The Global Tipping Points Report, University of Exeter, Exeter, UK, https://report-2023.global-tipping-points.org/download/4608/ (last access: 22 January 2025), 2023a.
Lenton, T. M., Xu, C., Abrams, J. F., Ghadiali, A., Loriani, S., Sakschewski, B., Zimm, C., Ebi, K. L., Dunn, R. R., Svenning, J. C., and Scheffer, M.: Quantifying the human cost of global warming, Nat. Sustain., 6, 1237–1247, https://doi.org/10.1038/s41893-023-01132-6, 2023b.
Leung, J. Y. S., Zhang, S., and Connell, S. D.: Is Ocean Acidification Really a Threat to Marine Calcifiers? A Systematic Review and Meta-Analysis of 980+ Studies Spanning Two Decades, Small, 18, 2107407, https://doi.org/10.1002/smll.202107407, 2022.
Li, G., Cheng, L., Zhu, J., Trenberth, K. E., Mann, M. E., and Abraham, J. P.: Increasing ocean stratification over the past half-century, Nat. Clim. Change, 10, 1116–1123, https://doi.org/10.1038/s41558-020-00918-2, 2020.
Liu, J., Luo, G., Lu, Z., Lu, W., Qie, W., Zhang, F., Wang, X., and Xie, S.: Intensified ocean deoxygenation during the end Devonian mass extinction, Geochem. Geophy. Geosy., 20, 6187–6198, https://doi.org/10.1029/2019GC008614, 2019.
Liu, J., Song, M., Zhu, Z., Horton, R. M., Hu, Y., and Xie, S.-P.: Arctic sea-ice loss is projected to lead to more frequent strong El Niño events, Nat. Commun., 13, 4952, https://doi.org/10.1038/s41467-022-32705-2, 2022.
Loeb, N. G., Johnson, G. C., Thorsen, T. J., Lyman, J. M., Rose, F. G., and Kato, S.: Satellite and ocean data reveal marked increase in Earth's heating rate, Geophys. Res. Lett., 48, e2021GL093047, https://doi.org/10.1029/2021GL093047, 2021.
Logan, C. A., Dunne, J. P., Ryan, J. S., Baskett, M. L., and Donner, S. D.: Quantifying global potential for coral evolutionary response to climate change, Nat. Clim. Change, 11, 537–542, 2021.
Lough, J. M., Anderson, K. D., and Hughes, T. P.: Increasing thermal stress for tropical coral reefs: 1871–2017, Sci. Rep.-UK, 8, 6079, https://doi.org/10.1038/s41598-018-24530-9, 2018.
MacNeil, M. A., Mellin, C., Matthews, S., Wolff, N. H., McClanahan, T. R., Devlin, M., Drovandi, C., Mengersen, K., and Graham, N. A.: Water quality mediates resilience on the Great Barrier Reef, Nat. Ecol. Evol., 3, 620–627, https://doi.org/10.1038/s41559-019-0832-3, 2019.
Maina, J., De Moel, H., Zinke, J., Madin, J., McClanahan, T., and Vermaat, J. E.: Human deforestation outweighs future climate change impacts of sedimentation on coral reefs, Nat. Commun., 4, 1–7, 2013.
Manzello, D. P., Brandt, M., Smith, T. B., Lirman, D., Hendee, J. C., and Nemeth, R. S.: Hurricanes benefit bleached corals, P. Natl. Acad. Sci. USA, 104, 12035–12039, 2007.
McClanahan, T. R.: Fisheries yields and species declines in coral reefs, Environ. Res. Lett., 17, 044023, https://doi.org/10.1088/1748-9326/ac5bb4, 2022.
McClanahan, T. R., Graham, N. A. J., MacNeil, M. A., and Cinner, J. E.: Biomass-based targets and the management of multispecies coral reef fisheries, Conserv. Biol., 29, 409–417, https://doi.org/10.1111/cobi.12430, 2015.
Meinshausen, M., Nicholls, Z. R. J., Lewis, J., Gidden, M. J., Vogel, E., Freund, M., Beyerle, U., Gessner, C., Nauels, A., Bauer, N., Canadell, J. G., Daniel, J. S., John, A., Krummel, P. B., Luderer, G., Meinshausen, N., Montzka, S. A., Rayner, P. J., Reimann, S., Smith, S. J., van den Berg, M., Velders, G. J. M., Vollmer, M. K., and Wang, R. H. J.: The shared socio-economic pathway (SSP) greenhouse gas concentrations and their extensions to 2500, Geosci. Model Dev., 13, 3571–3605, https://doi.org/10.5194/gmd-13-3571-2020, 2020.
Mellin, C., Brown, S., Cantin, N., Klein-Salas, E., Mouillot, D., Heron, S. F., and Fordham, D. A.: Cumulative risk of future bleaching for the world's coral reefs, Sci. Adv., 10, eadn9660, https://doi.org/10.1126/sciadv.adn9660, 2024.
Meyer, A. L. S., Bentley, J., Odoulami, R. C., Pigot, A. L., and Trisos, C. H.: Risks to biodiversity from temperature overshoot, Philos. T. Roy. Soc. B, B3772021039420210394, https://doi.org/10.1098/rstb.2021.0394, 2022.
Montgomery, A. D., Fenner, D., Donahue, M. J., and Toonen, R. J.: Community similarity and species overlap between habitats provide insight into the deep reef refuge hypothesis, Sci. Rep.-UK, 11, 23787, https://doi.org/10.1038/s41598-021-03128-8, 2021.
Muñiz-Castillo, A. I., Rivera-Sosa, A., Chollett, I., Eakin, C. M., Andrade-Gómez, L., McField, M., and Arias-González, J. E.: Three decades of heat stress exposure in Caribbean coral reefs: a new regional delineation to enhance conservation, Sci. Rep.-UK, 9, 11013, https://doi.org/10.1038/s41598-019-47307-0, 2019.
Naughten, K. A., Holland, P. R., and De Rydt, J.: Unavoidable future increase in West Antarctic ice-shelf melting over the twenty-first century, Nat. Clim. Change, 13, 1222–1228, https://doi.org/10.1038/s41558-023-01818-x, 2023.
Nelson, H. R. and Altieri, A. H.: Oxygen: the universal currency on coral reefs, Coral Reefs, 38, 177–198, https://doi.org/10.1007/s00338-019-01765-0, 2019.
Nicolet, K. J., Chong-Seng, K. M., Pratchett, M. S., Willis, B. L., and Hoogenboom, M. O.: Predation scars may influence host susceptibility to pathogens: evaluating the role of corallivores as vectors of coral disease, Sci. Rep.-UK, 8, 5258, https://doi.org/10.1038/s41598-018-23361-y, 2018.
Norström, A. V., Nyström, M., Jouffray, J. B., Folke, C., Graham, N., Moberg, A. J., Frederik, O., and Williams, G.: Guiding coral reef futures in the Anthropocene, Front. Ecol. Environ., 14, 490–498, https://doi.org/10.1002/fee.1427, 2016.
Obura, D., Gudka, M., Samoilys, M., Osuka, K., Mbugua, J., Keith, D. A., Porter, S., Roche, R., van Hooidonk, R., Ahamada, S., Araman, A., Karisa, J., Komakoma, J., Madi, M., Ravinia, I., Razafindrainibe, H., Yahya, S., and Zivane, F.: Vulnerability to collapse of coral reef ecosystems in the Western Indian Ocean, Nat. Sustain., 5, 104–113, https://doi.org/10.1038/s41893-021-00817-0, 2022.
OECD: Climate Tipping Points: Insights for Effective Policy Action, OECD Publishing, Paris, https://doi.org/10.1787/abc5a69e-en, 2022.
Packett, R., Dougall, C., Rohde, K., and Noble, R.: Agricultural lands are hot-spots for annual runoff polluting the southern Great Barrier Reef lagoon, Mar. Pollut. Bull., 58, 976–986, https://doi.org/10.1016/j.marpolbul.2009.02.017, 2009.
Perry, C. T., Murphy, G. N., Kench, P. S., Smithers, S. G., Edinger, E. N., Steneck, R. S., and Mumby, P. J.: Caribbean-wide decline in carbonate production threatens coral reef growth, Nat. Commun., 4, 1402, https://doi.org/10.1038/ncomms2409, 2013.
Perry, C. T., Alvarez-Filip, L., Graham, N. A., Mumby, P. J., Wilson, S. K., Kench, P. S., Manzello, D. P., Morgan, K. M., Slangen, A., Thomson, D. P., Januchowski-Hartley, F., Smithers, S. G., Steneck, R. S., Carlton, R., Edinger, E. N., Enochs, I. C., Estrada-Saldívar, N., Haywood, M. D. E., Kolodziej, G., Murphy, G. N., Pérez-Cervantes, E., Suchley, A., Valentino, L., Boenish, R., Wilson, M., and Macdonald, C.: Loss of coral reef growth capacity to track future increases in sea level, Nature, 558, 396–400, https://doi.org/10.1038/s41586-018-0194-z, 2018.
Pezner, A. K., Courtney, T. A., Barkley, H. C., Chou, W. C., Chu, H. C., Clements, S. M., Cyronak, T., DeGrandpre, M. D., Kekuewa, S. A., Kline, D. I., and Liang, Y. B.: Increasing hypoxia on global coral reefs under ocean warming, Nat. Clim. Change, 13, 403–409, 2023.
Plaisance, L., Caley, M. J., Brainard, R. E., and Knowlton, N.: The Diversity of Coral Reefs: What Are We Missing?, PLoS One, 6, e25026, https://doi.org/10.1371/journal.pone.0025026, 2011.
Prouty, N. G., Cohen, A., Yates, K. K., Storlazzi, C. D., Swarzenski, P. W., and White, D.: Vulnerability of coral reefs to bioerosion from land-based sources of pollution, J. Geophys. Res.-Oceans, 122, 9319–9331, 2017.
Puotinen, M., Drost, E., Lowe, R., Depczynski, M., Radford, B., Heyward, A., and Gilmour, J.: Towards modelling the future risk of cyclone wave damage to the world's coral reefs, Glob. Change Biol., 26, 4302–4315, 2020.
Randall, C. J. and van Woesik, R.: Contemporary white-band disease in Caribbean corals driven by climate change, Nat. Clim. Change, 5, 375–379, https://doi.org/10.1038/nclimate2530, 2015.
Raven, J., Caldeira, K., Elderfield, H., Hoegh-Guldberg, O., Liss, P., Riebesell, U., Shepherd, J., Turley, C., and Watson, A.: Ocean acidification due to increasing atmospheric carbon dioxide, The Royal Society, ISBN 0 85403 617 2, 2005.
Redding, J. E., Myers-Miller, R. L., Baker, D. M., Fogel, M., Raymundo, L. J., and Kim, K.: Link between sewage-derived nitrogen pollution and coral disease severity in Guam, Mar. Pollut. Bull., 73, 57–63, 2013.
Reed, D. C. and Harrison, J. A.: Linking nutrient loading and oxygen in the coastal ocean: A new global scale model, Global Biogeochem. Cy., 30, 447–459, https://doi.org/10.1002/2015GB005303, 2016.
Richards, Z. T., Juszkiewicz, D. J., and Hoggett, A.: Spatio-temporal persistence of scleractinian coral species at Lizard Island, Great Barrier Reef, Coral Reefs, 40, 1369–1378, https://doi.org/10.1007/s00338-021-02144-4, 2021.
Ripple, W. J., Wolf, C., Lenton, T. M., Gregg, J. W., Natali, S. M., Duffy, P. B., Rockström, J., and Schellnhuber, H. J.: Many risky feedback loops amplify the need for climate action, One Earth, 6, 86–91, 2023.
Rocha, J., Yletyinen, J., Biggs, R., Blenckner, T., and Peterson, G.: Marine regime shifts: drivers and impacts on ecosystems services, Philos. T. Roy. Soc. B, 370, 20130273, https://doi.org/10.1098/rstb.2013.0273, 2015.
Rocha, J. C., Peterson, G. D., Bodin, O., and Levin, S.: Cascading regime shifts within and across scales, Science, 362, 1379–1383, https://doi.org/10.1126/science.aat7850, 2018.
Rockström, J., Gupta, J., Lenton, T. M., Qin, D., Lade, S. J., Abrams, J. F., Jacobson, L., Rocha, J. C., Zimm, C., Bai, X., Bala, G. Bringezu, S., Broadgate, W., Bunn, S. E., DeClerck, F., Ebi, K. E., Gong, P., Gordon, C., Kanie, N., Liverman, D. M., Nakicenovic, N., Obura, D., Ramanathan, V., Verburg, P. H., van Vuuren, D. P., and Winkelmann, R.: Identifying a safe and just corridor for people and the planet, Earths Future, 9, e2020EF001866, https://doi.org/10.1029/2020EF001866, 2021.
Rodrigues, L. J. and Padilla-Gamiño, J. L.: Trophic provisioning and parental trade-offs lead to successful reproductive performance in corals after a bleaching event, Sci. Rep.-UK, 12, 18702, https://doi.org/10.1038/s41598-022-21998-4, 2022.
Sabine, C. L., Feely, R. A., Gruber, N., Key, R. M., Lee, K., Bullister, J. L., Wanninkhof, R., Wong, C. S. L., Wallace, D. W., Tilbrook, B., Millero, F. J., Peng, T. H., Kozyr, A., Ono, T., and Rios, A. F.: The oceanic sink for anthropogenic CO2, Science, 305, 367–371, 2004.
Saintilan, N., Horton, B., Törnqvist, T. E., Ashe, E. L., Khan, N. S., Schuerch, M., Perry, C., Kopp, R. E., Garner, G. G., Murray, N., Rogers, K., Albert, S., Kelleway, J., Shaw, T. A., Woodroffe, C. D., Lovelock, C. E., Goddard, M. M., Hutley, L. B., Kovalenko, K., Feher, L., and Guntenspergen, G.: Widespread retreat of coastal habitat is likely at warming levels above 1.5 °C, Nature, 621, 112–119, https://doi.org/10.1038/s41586-023-06448-z, 2023.
Saunders, M., Leon, J., Callaghan, D. P., Roelfsema, C. M., Hamylton, S., Brown, C. J., Baldock, T., Golshani, A., Phinn, S. R., Lovelock, C. E., Hoegh-Guldberg, O., Colin, D., Woodroffe, C. D., and Mumby, P. J.: Interdependency of tropical marine ecosystems in response to climate change, Nat. Clim. Change, 4, 724–729, https://doi.org/10.1038/nclimate2274, 2014.
Saunders, M. I., Albert, S., Roelfsema, C. M., Leon, J. X., Woodroffe, C. D., Phinn, S. R., and Mumby, P. J.: Tectonic subsidence provides insight into possible coral reef futures under rapid sea-level rise, Coral Reefs, 35, 155–167, https://doi.org/10.1007/s00338-015-1365-0, 2016.
Schleussner, C. F., Ganti, G., Lejeune, Q., Zhu, B., Pfleiderer, P., Prütz, R., Ciais, P., Frölicher, T. L., Fuss, S., Gasser, T., Gidden, M. J., Kropf, C. M., Lacroix, F., Lamboll, R., Martyr, R., Maussion, F., McCaughey, J. W., Meinshausen, M., Mengel, M., Nicholls, Z., Quilcaille, Y., Sanderson, B., Seneviratne, S. I., Sillmann, J., Smith, C. J., Steinert, N. J., Theokritoff, E.,Warren, R., Price, J., and Rogelj, J.: Overconfidence in climate overshoot, Nature, 634, 366–373, https://doi.org/10.1038/s41586-024-08020-9, 2024.
Setter, R. O., Franklin E. C., and Mora, C.: Co-occurring anthropogenic stressors reduce the timeframe of environmental viability for the world's coral reefs, PLoS Biol., 20, e3001821, https://doi.org/10.1371/journal.pbio.3001821, 2022.
Sheppard, C., Sheppard, A., and Fenner, D.: Coral mass mortalities in the Chagos Archipelago over 40 years: Regional species and assemblage extinctions and indications of positive feedbacks, Mar. Pollut. Bull., 154, 111075, https://doi.org/10.1016/j.marpolbul.2020.111075, 2020.
Sheppard, C. R. C. and Sheppard, A. L. S.: British Indian Ocean Territory (Chagos Archipelago), in: World Seas: An environmental assessment, vol. 3, edited by: Sheppard, C. R. C., Academic Press, 237–252, https://doi.org/10.1016/B978-0-08-100853-9.00015-4, 2019.
Simpson, C. J., Cary, J. L., and Masini, R. J.: Destruction of corals and other reef animals by coral spawn slicks on Ningaloo Reef, Western Australia, Coral Reefs, 12, 185–191, 1993.
Skirving, W. J., Heron, S. F., Marsh, B. L., Liu, G., De La Cour, J. L., Geiger, E. F., and Eakin, C. M.: The relentless march of mass coral bleaching: a global perspective of changing heat stress, Coral Reefs, 38, 547–557, https://doi.org/10.1007/s00338-019-01799-4, 2019.
Souter, D., Planes, S., Wicquart, J., Logan, M., Obura, D., and Staub, F. (Eds.): Status of coral reefs of the world: 2020 report, Global Coral Reef Monitoring Network (GCRMN) and International Coral Reef Initiative (ICRI), https://doi.org/10.59387/WOTJ9184, 2021.
Spada, G., Bamber, J. L., and Hurkmans, R. T. W. L.: The gravitationally consistent sea-level fingerprint of future terrestrial ice loss, Geophys. Res. Lett., 40, 482–486, https://doi.org/10.1029/2012GL053000, 2013.
Speare, K. E., Adam, T. C., Winslow, E. M., Lenihan, H. S., and Burkepile, D. E.: Size-dependent mortality of corals during marine heatwave erodes recovery capacity of a coral reef, Glob. Change Biol,, 28, 1342–1358, https://doi.org/10.1111/gcb.16000, 2022.
Suwa, R., Nakamura, M., Morita, M., Shimada, K., Iguchi, A., Sakai, K., and Suzuki, A.: Effects of acidified seawater on early life stages of scleractinian corals (Genus Acropora), Fisheries Sci., 76, 93–99, 2010.
Tachiiri, K., Herran, D. S., Su, X., and Kawamiya, M.: Effect on the Earth system of realizing a 1.5 °C warming climate target after overshooting to the 2 °C level, Environ. Res. Lett., 14, 124063, https://doi.org/10.1088/1748-9326/ab5199, 2019.
Tanaka K. R. and Van Houtan K. S.: The recent normalization of historical marine heat extremes, PLOS Clim., 1, e0000007, https://doi.org/10.1371/journal.pclm.0000007, 2022.
Thinesh, T., Meenatchi, R., Pasiyappazham, R., Jose, P. A., Selvan, M., Kiran, G. S., and Selvin, J.: Short-term in situ shading effectively mitigates linear progression of coral-killing sponge Terpios hoshinota, PLoS One, 12, e0182365, https://doi.org/10.1371/journal.pone.0182365, 2017.
Thurber, R. V., Mydlarz, L. D., Brandt, M., Harvell, D., Weil, E., Raymundo, L., Willis, B. L., Langevin, S., Tracy, A. M., Littman, R., Kemp, K. M., Dawkins, P., Prager, K. C., Garren, M., and Lamb, J.: Deciphering coral disease dynamics: Integrating host, microbiome, and the changing environment, Front. Ecol. Evol., 30, 575927, https://doi.org/10.3389/fevo.2020.575927, 2020.
Törnqvist, T. E., Cahoon, D. R., Morris, J. T., and Day, J. W.: Coastal wetland resilience, accelerated sea-level rise, and the importance of timescale, AGU Advances, 2, e2020AV000334, https://doi.org/10.1029/2020AV000334, 2021.
Toth, L. T., Precht, W. F., Modys, A. B., Stathakopoulos, A., Robbart, M. L., Hudson, J. H., Oleinik, A. E., Riegl, B. M., Shinn, E. A., and Aronson, R. B.: Climate and the latitudinal limits of subtropical reef development, Sci. Rep.-UK, 11, 13044, https://doi.org/10.1038/s41598-021-87883-8, 2021.
UNEP: Projections of future coral bleaching conditions using IPCC CMIP6 models: climate policy implications, management applications, and Regional Seas summaries, United Nations Environment Programme, Nairobi, Kenya, https://wedocs.unep.org/20.500.11822/34219 (last access: 27 January 2025) 2020.
Uthicke, S., Logan, M., Liddy, M., Francis, D., Hardy, N., and Lamare, M.: Climate change as an unexpected co-factor promoting coral eating seastar (Acanthaster planci) outbreaks, Sci. Rep.-UK, 5, 8402, https://doi.org/10.1038/srep08402, 2015.
Van Hooidonk, R., Maynard, J. A., Manzello, D., and Planes, S.: Opposite latitudinal gradients in projected ocean acidification and bleaching impacts on coral reefs, Glob. Change Biol., 20, 103–112, https://doi.org/10.1111/gcb.12394, 2014.
Vaquer-Sunyer, R. and Duarte, C. M.: Temperature effects on oxygen thresholds for hypoxia in marine benthic organisms, Glob. Change Biol., 17, 1788–1797, 2011.
Venegas, R. M., Acevedo, J., and Treml, E. A.: Three decades of ocean warming impacts on marine ecosystems: A review and perspective, Deep-Sea Res. Pt. II, 212, 105318, https://doi.org/10.1016/j.dsr2.2023.105318, 2023.
Vercelloni, J., Caley, M. J., and Mengersen, K. L.: Thresholds of Coral Cover that Support Coral Reef Biodiversity, in: Case Studies in Applied Bayesian Data Science, edited by: Mengersen, K., Pudlo, P., and Robert, C., Lect. Notes Math., vol. 2259, Springer, Cham, https://doi.org/10.1007/978-3-030-42553-1_16, 2020.
Vernimmen, R. and Hooijer, A.: New LiDAR-Based Elevation Model Shows Greatest Increase in Global Coastal Exposure to Flooding to Be Caused by Early-Stage Sea-Level Rise, Earths Future, 11, e2022EF002880, https://doi.org/10.1029/2022EF002880, 2023.
Veron, J. E. N., Hoegh-Guldberg, O., Lenton, T. M., Lough, J. M., Obura, D. O., Pearce-Kelly, P., Sheppard, C. R. C., Spalding, M., Stafford-Smith, M. G., and Rogers, A. D.: The coral reef crisis: The critical importance of <350 ppm CO2, Mar. Pollut. Bull., 58, 1428–1436, 2009.
von Schuckmann, K., Cheng, L., Palmer, M. D., Hansen, J., Tassone, C., Aich, V., Adusumilli, S., Beltrami, H., Boyer, T., Cuesta-Valero, F. J., Desbruyères, D., Domingues, C., García-García, A., Gentine, P., Gilson, J., Gorfer, M., Haimberger, L., Ishii, M., Johnson, G. C., Killick, R., King, B. A., Kirchengast, G., Kolodziejczyk, N., Lyman, J., Marzeion, B., Mayer, M., Monier, M., Monselesan, D. P., Purkey, S., Roemmich, D., Schweiger, A., Seneviratne, S. I., Shepherd, A., Slater, D. A., Steiner, A. K., Straneo, F., Timmermans, M.-L., and Wijffels, S. E.: Heat stored in the Earth system: where does the energy go?, Earth Syst. Sci. Data, 12, 2013–2041, https://doi.org/10.5194/essd-12-2013-2020, 2020.
von Schuckmann, K., Minière, A., Gues, F., Cuesta-Valero, F. J., Kirchengast, G., Adusumilli, S., Straneo, F., Ablain, M., Allan, R. P., Barker, P. M., Beltrami, H., Blazquez, A., Boyer, T., Cheng, L., Church, J., Desbruyeres, D., Dolman, H., Domingues, C. M., García-García, A., Giglio, D., Gilson, J. E., Gorfer, M., Haimberger, L., Hakuba, M. Z., Hendricks, S., Hosoda, S., Johnson, G. C., Killick, R., King, B., Kolodziejczyk, N., Korosov, A., Krinner, G., Kuusela, M., Landerer, F. W., Langer, M., Lavergne, T., Lawrence, I., Li, Y., Lyman, J., Marti, F., Marzeion, B., Mayer, M., MacDougall, A. H., McDougall, T., Monselesan, D. P., Nitzbon, J., Otosaka, I., Peng, J., Purkey, S., Roemmich, D., Sato, K., Sato, K., Savita, A., Schweiger, A., Shepherd, A., Seneviratne, S. I., Simons, L., Slater, D. A., Slater, T., Steiner, A. K., Suga, T., Szekely, T., Thiery, W., Timmermans, M.-L., Vanderkelen, I., Wjiffels, S. E., Wu, T., and Zemp, M.: Heat stored in the Earth system 1960–2020: where does the energy go?, Earth Syst. Sci. Data, 15, 1675–1709, https://doi.org/10.5194/essd-15-1675-2023, 2023.
Walker, A. S., Kratochwill, C. A., and van Woesik, R.: Past disturbances and local conditions influence the recovery rates of coral reefs, Glob. Change Biol., 30, e17112, https://doi.org/10.1111/gcb.17112, 2024.
Wang, S., Foster, A., Lenz, E. A., Kessler, J. D., Stroeve, J. C., Anderson, L. O., Turetsky, M., Betts, R., Zou, S., Liu, W., Boos, W. R., and Hausfather, Z.: Mechanisms and impacts of Earth system tipping elements, Rev. Geophys., 61, e2021RG000757, https://doi.org/10.1029/2021RG000757, 2023.
Weber, M., De Beer, D., Lott, C., Polerecky, L., Kohls, K., Abed, R. M., Ferdelman, T. G., and Fabricius, K. E.: Mechanisms of damage to corals exposed to sedimentation, P. Natl. Acad. Sci. USA, 109, 1558–1567, 2012.
Woodroffe, C. D. and Webster, J. M.: Coral reefs and sea-level change, Mar. Geol., 352, 248–267, https://doi.org/10.1016/j.margeo.2013.12.006, 2014.
Wunderling, N., Winkelmann, R., Rockström, J., Loriani, S., Armstrong-McKay, D., Ritchie, P., Sakschewski, B., and Donges, J.: Global warming overshoots increase risks of climate tipping cascades in a network model, Nat. Clim. Change, 13, 75–82, https://doi.org/10.1038/s41558-022-01545-9, 2023.
Zhang, Z., Poulter, B., Feldman, A. F., Ying, Q., Ciais, P., Peng, S., and Li, X.: Recent intensification of wetland methane feedback, Nat. Clim. Change, 13, 430–433, https://doi.org/10.1038/s41558-023-01629-0, 2023.
Zuo, X., Su, F., Yu, K., Wang, Y., Wang, Q., and Wu, H.: Spatially Modeling the Synergistic Impacts of Global Warming and Sea-Level Rise on Coral Reefs in the South China Sea, Remote Sens.-Basel, 13, 2626, https://doi.org/10.3390/rs13132626, 2021.
- Abstract
- Introduction
- Considerations for assessing coral reef TPs
- Ocean warming and heatwaves
- Stratification
- Ocean acidification
- Deoxygenation
- Storm intensity
- Sea level rise
- Pollution and disruption
- Disease
- Invasive and other problem species
- Reef impact example
- Cascade effects contributing to coral reef TP threshold sensitivity
- Resilience, adaptation, and refugia
- Conclusions
- Data availability
- Author contributions
- Competing interests
- Disclaimer
- Special issue statement
- Acknowledgements
- Financial support
- Review statement
- References
- Abstract
- Introduction
- Considerations for assessing coral reef TPs
- Ocean warming and heatwaves
- Stratification
- Ocean acidification
- Deoxygenation
- Storm intensity
- Sea level rise
- Pollution and disruption
- Disease
- Invasive and other problem species
- Reef impact example
- Cascade effects contributing to coral reef TP threshold sensitivity
- Resilience, adaptation, and refugia
- Conclusions
- Data availability
- Author contributions
- Competing interests
- Disclaimer
- Special issue statement
- Acknowledgements
- Financial support
- Review statement
- References