the Creative Commons Attribution 4.0 License.
the Creative Commons Attribution 4.0 License.
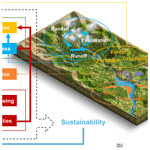
Coupling human and natural systems for sustainability: experience from China's Loess Plateau
Bojie Fu
Xutong Wu
Zhuangzhuang Wang
Xilin Wu
Shuai Wang
Addressing the sustainability challenges that humanity is facing in the Anthropocene requires the coupling of human and natural systems, rather than their separate treatment. To help understand the dynamics of a coupled human and natural system (CHANS) and support the design of policies and measures that promote sustainability, we propose a conceptual cascade framework of “pattern–process–service–sustainability”, which is characterized by coupling landscape patterns and ecological processes, linking ecological processes to ecosystem services, and promoting social–ecological sustainability. The use of this framework is illustrated by a review of CHANS research experience in China's Loess Plateau (LP), a well-known region for its historically severe soil erosion and successful ecological restoration achieved in recent decades. Ecological restoration in the LP has greatly increased its vegetation coverage and controlled its soil erosion. However, some accompanied issues, like soil drying in some areas due to the introduction of exotic plant species and the mismanagement of planted vegetation and water use conflicts between vegetation and humans caused by the trade-off between carbon sequestration and water supply, have started to threaten the long-term sustainability of the LP. Based on a comprehensive understanding of CHANS dynamics, the social–ecological sustainability of the LP can be improved through enhancing water and food security, implementing basin-wide governance, maintaining ecological restoration achievements, and promoting rural livelihood transition. The research experience accumulated on the LP offers examples of the application of the pattern–process–service–sustainability framework. Future research using this framework should especially focus on the integrated research of multiple processes; the cascades of ecosystem structure, function, services, and human well-being; the feedback mechanisms of human and natural systems; and the data and models for sustainability.
- Article
(6375 KB) - Full-text XML
- BibTeX
- EndNote
In the Anthropocene, accelerated landscape modification and climate change are altering the earth's surface processes, leading to a series of social and environmental issues (Steffen et al., 2007; Lewis and Maslin, 2015). Climate change, forest loss, ecosystem degradation, and the depletion of resources are global concerns, threatening the sustainability of human society (Intergovernmental Science-Policy Platform on Biodiversity and Ecosystem Services, 2019; MA, 2005). Addressing human society toward a path of sustainable development has become an urgent item on the research agenda of the scientific community and policymakers (UN, 2015). To promote international cooperation on sustainable development, in 2000 the UN Millennium Declaration agreed on the eight Millennium Development Goals, and in 2015 the launch of the 2030 Agenda by the UN announced the 17 Sustainable Development Goals (SDGs). However, recent reports suggested that it may not be possible to achieve the SDGs by 2030 (UN, 2019), mainly due to the complex and intertwined nature of these pressing sustainability challenges that human beings face (Biggs et al., 2021). In recent decades, a growing consensus in academia has been reached, asserting that sustainability challenges cannot be properly addressed until humans and nature are treated as an entirety (Biggs et al., 2021; Cumming, 2014).
The coupled human and natural systems (CHANSs), which are also referred to as coupled human and Earth systems, human and environment systems, and social–ecological systems, describe a complex phenomenon in which human processes and natural processes interact and co-evolve (Wang et al., 2018b; Quintas-Soriano et al., 2021; Ostrom, 2009; Liu et al., 2009; Fu and Wei, 2018; Cumming, 2014). In CHANSs, humans alter the ecosystem patterns and processes to obtain desired ecosystem services, such as food, fresh water, and timber; natural processes can affect human systems through environmental degradation and disasters, such as soil erosion, floods, and droughts (Wang et al., 2018b; Quintas-Soriano et al., 2021; Ostrom, 2009; Liu et al., 2009; Fu and Wei, 2018; Cumming, 2014). CHANSs provide a holistic and integrated perspective to combine the human and natural dimensions and elucidate their complex feedback mechanisms (J. Liu et al., 2013; Tian, 2017; Partelow, 2018), which is needed to develop innovative insights and solutions to the sustainability challenges that characterize the Anthropocene (Liu et al., 2021). As CHANSs involve multiple natural and human processes interacting at different scales, this complexity poses a challenge for researchers to understand their dynamics (Liu et al., 2007; Nelson et al., 2007; Rocha et al., 2015; Gunderson et al., 2017). However, research paradigms that explicitly guide sustainability practices based on deepened understandings of the interactions between humans and nature are still limited. To fill this knowledge gap, we propose a conceptual cascade framework of “pattern–process–service–sustainability” (Fu and Wei, 2018) to better observe, analyse, and understand the dynamics of CHANSs and promote their sustainability.
This framework provides instructive steps to study CHANSs and support sustainability practices by coupling landscape patterns and ecological processes, linking ecological processes to ecosystem services, and promoting social–ecological sustainability. Pattern–process coupling provides a critical approach for integrated research and a comprehensive understanding of the mechanisms of natural processes, such as the carbon–water cycle, ecohydrological processes, and soil–vegetation–atmosphere interactions (Fu et al., 2019). Ecosystem services (ESs) are the benefits that people directly or indirectly obtain from ecosystems, and their changes will affect human well-being and sustainable development (Costanza et al., 2017). Ecological processes and functions transform into services when they can (directly or indirectly) benefit humans (Costanza et al., 2017). Unlike the research on pattern–process coupling, ES research places a greater emphasis on the social dimension or human needs of ecosystems. Managing ecosystem processes to sustain the provision of ESs is the basic way to improve sustainability (Fu et al., 2013), constituting an important bridge between science and policy. For example, through land use planning and management, the land use structure can be optimized to obtain the desired and sustainable provision of ESs. To elucidate the complex feedback mechanism of CHANSs, it is necessary to couple patterns and processes and link processes and services (Wang et al., 2018b), and the findings obtained could support policy-related decisions that enhance sustainability.
This paper starts with a detailed introduction of the proposed framework and its components, and then it systematically illustrates the use of this framework to study the dynamics of CHANSs and support decision making for the promotion of sustainability through a review of research experience in China's Loess Plateau (LP). Over the last 20 years, researchers have conducted extensive studies on the landscape patterns, ecological processes, and ESs of the LP, making it an ideal region for summarizing research experience and providing guidance for the CHANS studies. Finally, several future directions of the pattern–process–service–sustainability framework are proposed by revealing the limitations of current studies.
The proposed pattern–process–service–sustainability framework (Fig. 1) provides a paradigm for researchers to study CHANSs and identify practical solutions for adaptation to environmental change (Fu and Wei, 2018). The Earth's surface landscape has been extensively shaped and modified by natural forces and human activities, which are reflected in the pattern of landscape components (Steffen et al., 2015, 2007). The term “pattern” generally refers to a spatial pattern of landscape components, including their properties of size, type, number, and spatial distribution, and the term “process” indicates the dynamic features of the occurrence and evolution of incidents or phenomena (Fu et al., 2011a). Patterns and processes interact with each other, which can be expressed as “patterns influence processes, and processes change patterns” (Fu et al., 2011a, 2019). For example, land use configurations can influence soil erosion processes, and hydrological processes, such as floods, can quickly alter the land cover pattern of alluvial plains. These reciprocal effects between patterns and processes are critical to understanding the coupling mechanisms of CHANSs (Fu and Wei, 2018).
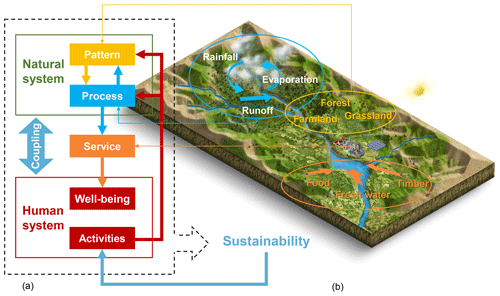
Figure 1Illustration of the pattern–process–service–sustainability framework. A flowchart of the pattern, process, service, and sustainability framework in the coupled human and natural system (a) and a diagram of typical patterns, processes (e.g. hydrological processes), and ESs (e.g. provision services) (b).
ESs are defined as the ecosystem characteristics, processes, or functions that directly or indirectly contribute to human well-being (Costanza et al., 2017). A better understanding of ESs and especially the links between them and ecological processes is crucial to accurately assess and predict ES dynamics. The relationships between ecological processes and ESs are complex: multiple ESs may share the same ecological process, or multiple ecological processes may support the same ES (Bennett et al., 2009; Fu et al., 2013). In addition, ecological processes interact with each other, rather than exist independently (Fu et al., 2015). For instance, both soil conservation and water yield services are affected by rainfall events, runoff, and vegetation dynamics. Therefore, changes in ecological patterns and processes caused by both natural forces and human activities can affect multiple ESs through cascade effects, resulting in trade-offs and synergy effects between services (Fu et al., 2013). Specifically, a trade-off occurs when one service increases and another one decreases, while synergy indicates the presence of the same variation trend for both services (Bennett et al., 2009). Only when ecological processes and ESs are considered together is it possible to comprehensively understand the driving mechanisms of ESs and identify the critical processes that could be regulated to optimize them (Fu et al., 2013).
The practical implications of CHANSs studies are to promote the sustainability of the coupled systems (Ostrom, 2009; J. Liu et al., 2013). A deep understanding of pattern–process–service interactions can help us figure out the causes of the unsustainable status of a CHANS and provide the scientific basis for designing policies or measures that promote its sustainability. Both environmental changes and human activities can affect the interactions between landscape patterns and ecological processes and directly or indirectly affect the ESs upon which humans depend (Fu et al., 2019). Targeted sustainability activities such as land use optimization provide feasible and effective ways to manage landscape patterns and ecosystem processes and to mitigate trade-offs and enhance synergies among ESs, which finally bring win–win gains between human and nature and improve the sustainability of CHANSs. Thus, the above-mentioned analytical paradigm for practical management can be integrated into a pattern–process–service–sustainability cascade framework (Fu and Wei, 2018). To use this paradigm for the promotion of CHANS sustainability, it is necessary to couple landscape patterns and ecological processes, link ecological processes to services, and bridge science and policy (Fu et al., 2015). In the following sections, research conducted in China's LP was used as an example to illustrate how this framework is applied to empirical studies.
The LP (Fig. 2), located in central China, is the largest and thickest loess deposit in the world, covering an area of 640 000 km2 (Fu et al., 2017). It is characterized by a continental monsoon climate with an average annual precipitation of 400 mm (Table 1), and most of the plateau is located in a semiarid zone, based on the aridity index (Chen et al., 2007). Because the loess is highly fertile and easy to cultivate (Wang et al., 2010), early Chinese agriculture and civilization developed around the LP beginning in the Neolithic era (Barton et al., 2009; Sun et al., 2018). However, due to the anthropic impacts affecting the area for thousands of years, the LP has suffered from soil erosion, land degradation, natural disasters, and extreme poverty (Fu et al., 2017). These undesired social and ecological outcomes resulted from the mutually reinforcing feedback between natural and human processes (Wu et al., 2020).
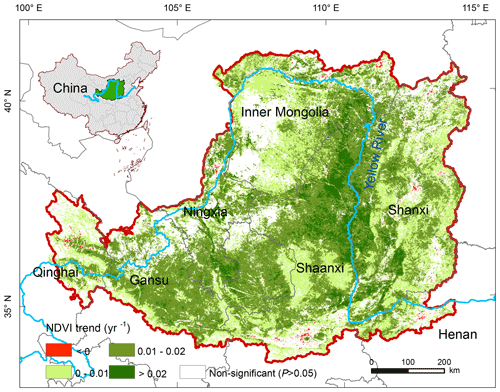
Figure 2Location of the Loess Plateau and its normalized difference vegetation index (NDVI) trend from 2000 to 2015.
The erosion susceptibility of loess, periodic extreme rainfall events, sparse vegetation, and long-term and intensive farming activities shaped the fragile ecological environment on the LP, exacerbating the sensitivity of the region to extreme climatic events and human disturbances (Fu et al., 2017). As the human population increased, more deforestation and land reclamation occurred to meet the growing demand for food and firewood. Long-term human-induced vegetation degradation also exacerbated soil erosion, turning more than 70 % of the once flat plateau into a region dominated by hills and gullies (Zhao et al., 2013). In addition, the Yellow River, the second largest river in China, flows through the LP and receives nearly 90 % of the sediment load from this region (Wang et al., 2016). Due to a large amount of sediment deposited in the lower reaches of the river, frequent flood and breach events have historically occurred, causing huge losses to the residents living in the alluvial plain (Chen et al., 2012; Chen, 2019). Furthermore, severe soil erosion caused a decline in land productivity and grain yield, and therefore more land needed to be reclaimed to feed the growing population (Chen et al., 2007). This vicious cycle seems to have determined the “fall” of the LP into a social–ecological trap.
To break out of this negative cycle, the Chinese government has taken numerous efforts to restore vegetation and control soil erosion in the region, in order to establish a sustainable development path. Since the 1970s, numerous soil and water conservation measures have been implemented, including the construction of terracing and check dams, integrated watershed management for soil and water conservation, and vegetation restoration (Fu et al., 2017; Yu et al., 2020). Among them, the Grain-to-Green Program (GTGP), implemented in 1999 in the LP, is the largest and most successful (Feng et al., 2016; Wu et al., 2020). Since the beginning of the 21st century, the vegetation coverage in the LP has significantly increased and soil erosion has been effectively controlled (Fu et al., 2011b). According to the MODIS (Moderate Resolution Imaging Spectroradiometer) NDVI data, more than 70 % of the LP showed a significant “greening” trend from 2000 to 2015, mainly distributed in the central and southern parts (Fig. 2).
Despite the success of the GTGP, many uncertainties remain in relation to the effects of ecological restoration on social–ecological sustainability (Fu et al., 2017), whose achievement in the LP has become the focus for researchers and policymakers. In the last 2 decades, extensive studies have been conducted on the CHANS of the LP to better understand its multiple natural and human processes and to support policy measures for ecological restoration (Feng et al., 2010; Fu et al., 2011a, 2017). The representative social–ecological context of the LP and the extensive studies make it an ideal region for summarizing the research experience of CHANS. In the following section, a review of CHANS studies conducted on the LP using the pattern–process–service–sustainability framework is reported.
4.1 Coupling patterns and processes
Through a process of natural evolution, the atmosphere–soil–vegetation interaction reaches a dynamic equilibrium, forming a relatively stable vegetation pattern (Zhang et al., 2018). However, deforestation and afforestation can alter this equilibrium and cause a series of ecohydrological effects that influence water, carbon, soil processes, and the overall ecosystem sustainability (Feng et al., 2016; Zhang et al., 2018). The GTGP, launched in 1999, converted slope croplands into forest or grasslands, thus altering the landscape pattern in the LP (Fu et al., 2011b). Therefore, understanding the ecohydrological effects caused by vegetation restoration through the coupling of patterns and processes is of great significance to direct subsequent ecological restoration measures (Fu et al., 2011a). In order to achieve such an understanding, researchers have conducted numerous studies on soil erosion processes, carbon–water processes (Feng et al., 2016), and flow–sediment relationships in the LP (Wang et al., 2016).
In the context of ecological restoration, the pressing task is to understand the soil erosion dynamics in the LP and what land use pattern will result in the best soil and water conservation effect. Based on long-term field monitoring and control experiments (such as the building of runoff plots), studies have found that rainfall events characterized by a high intensity, short duration, and high frequency had the most erosive effect (Wei et al., 2007), and the most severe annual erosion rates appeared in drought years, rather than in wet years (Wei et al., 2010); therefore, more attention should be paid to rainfall variability and distribution to understand soil erosion processes in the LP. For vegetation patterns, shrubs are a superior choice to control soil erosion compared to other vegetation types (Wei et al., 2007; Zhou et al., 2016) because of their differences in canopy structure and surface litter layer (Zhou et al., 2016). Comparative experiments confirmed that soil compaction processes occurred during revegetation, which resulted in the increase of runoff and a non-significant decrease of soil erosion in runoff plots, even though vegetation increased (Liu et al., 2012). Furthermore, the combination of plant traits, such as a small mean root diameter and great root tensile strength, can exert the greatest soil erosion control effect (Zhu et al., 2015). Overall, the main drivers of soil erosion in the LP include the characteristics of precipitation (e.g. intensity and duration), vegetation patterns, soil properties, and vegetation traits, which could inspire effective practices to prevent soil erosion (Zhou et al., 2016).
Ecological restoration in the LP altered the carbon and water cycles, even creating a severe carbon–water conflict (Fig. 3). After the implementation of the GTGP, the NPP (net primary productivity) and NEP (net ecosystem productivity) have steadily increased, and a total of 96.1 Tg of additional carbon was sequestered from 2000 to 2008, transforming the LP from a net carbon source to a net carbon sink (Feng et al., 2013). However, the introduced vegetation will inevitably consume a large amount of water resources, causing a conflict between plant growth and water consumption. Numerous field monitoring and experiments have reported that soil moisture decline and soil desiccation occurred in the LP due to vegetation restoration (Chen et al., 2010; Liang et al., 2018; Wang et al., 2013; Yao et al., 2012). In addition, the remote sensing and model-based approach reached similar conclusions, namely that overplanting is responsible for soil drying in the LP (Zhang et al., 2018). Based on currently revegetated areas and water demand for human consumption, Feng et al. (2016) estimated a threshold of NPP of 400±5 in the LP, above which the population will suffer water shortages, and the results indicated that the NPP in these revegetated areas was close to this limit. Future climate change will affect this permissible NPP threshold. Considering the maximum, median, and minimum scenarios of precipitation change and future changes in plant water-use efficiency, permissible NPP by 2050 is 578±48, 473±41, and 309±29 , respectively (Feng et al., 2016). Moreover, Zhang et al. (2018) estimated the equilibrium vegetation cover over the LP based on an ecohydrological model and ecosystem optimality theory (i.e. minimum water stress assumption) and compared it with the actual vegetation cover obtained from remote sensing data. The results showed that the current vegetation cover (0.48 on average) has already exceeded the climate-defined equilibrium vegetation cover (0.43 on average) in many parts of the LP, especially in the central and eastern regions (Zhang et al., 2018). In addition to excessive vegetation density, the types of introduced vegetation can also have a great impact on soil drying. Field experiments showed that soil moisture conditions were better where native species, rather than exotic species, grew. For example, pine and Robinia pseudoacacia plantations may not be appropriate for ecological restoration in a semi-arid loess hilly area such as the LP due to the high water consumption and poor water retention of these plants (Chen et al., 2010; Liang et al., 2018). Even land abandonment is a more effective method to maintain soil water than revegetation with introduced plants (Yao et al., 2012; An et al., 2017).
Runoff and sediment transport have a great impact on biogeochemical processes that determine ecosystem health in river basins (Gao et al., 2016, 2017). Understanding the dominant mechanism behind the variation of flow–sediment relationships is crucial to ensure sustainable water management (Gao et al., 2017). The LP used to contribute nearly 90 % of the Yellow River sediment (Wang et al., 2017). However, a sharp reduction (58 %) in the river sediment was detected after 1979 (Wang et al., 2017) (Fig. 3). Based on the relationship between cumulative sediment load and cumulative precipitation, this sediment reduction trend can be classified into three periods: 1951–1979 (1.35±0.65 Gt yr−1), 1980–1999 (0.73±0.28 Gt yr−1), and 2000–2010 (0.32±0.24 Gt yr−1) (Wang et al., 2016). To elucidate the complex driving mechanism of sediment load change, an attribution method was developed to analyse 60 years of runoff and sediment load observations from the traverse of the Yellow River over the LP (Wang et al., 2016). The results showed that human activities are the main cause of sediment reduction in the Yellow River. Specifically, landscape engineering, terracing, and the construction of check dams and reservoirs were the primary factors driving the sediment load reduction before 2000, accounting for 54 %, while after 2000, the main contributor was vegetation restoration, accounting for 57 % (Wang et al., 2016). Reduced runoff and sediment in the Yellow River can produce a series of ecological and socioeconomic effects. For instance, sediment reduction can improve the water quality, but it can also lead to the shrinking of the Yellow River delta, and inadequate environmental flows may affect aquatic habitats (Wu et al., 2020).
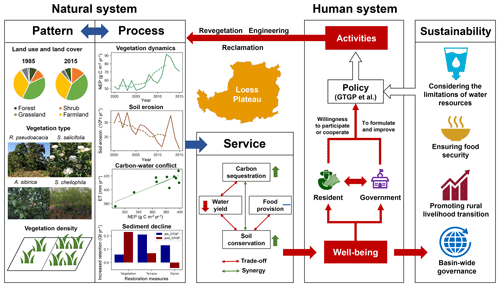
Figure 3Illustration of the coupled human and natural system studies of China's Loess Plateau using the pattern–process–service–sustainability framework. Data used in this figure were obtained from Wang et al. (2016), Feng et al. (2016), Liu et al. (2019), and Wu et al. (2019).
In summary, the studies on soil erosion and water–carbon processes, and on flow–sediment changes after vegetation restoration in the LP (Feng et al., 2016; Gao et al., 2016; Wang et al., 2016; Zhou et al., 2016) are of great significance for the establishment of future vegetation restoration measures. However, there are still some limitations in current studies. First, the coupling level of multiple ecological processes is still insufficient. For example, soil erosion is simultaneously affected by multiple natural and anthropogenic factors, including precipitation, terrain, soil properties, land use and land cover types, and even vegetation root traits (Zhou et al., 2016; Zhu et al., 2015). The current studies in the LP mainly coupled only two or three processes, such as precipitation and land use and land cover types (Zhou et al., 2016), which may increase the uncertainty of the results. Second, as the upper, middle, and lower reaches of the Yellow River are interrelated, ecological restoration carried out in the LP area located in its middle reaches will inevitably influence the areas in the lower reaches; however, a basin-wide perspective to study the ecohydrological effects caused by ecological restoration in the LP is still lacking (Wang et al., 2017).
4.2 Linking ecological processes to ESs
By coupling patterns and processes, researchers found that the ecological restoration programme has altered the soil erosion and water–carbon processes and flow–sediment relationships in the LP (Feng et al., 2016; Wang et al., 2016). As ecological processes underpin the delivery of ESs, changes in these natural processes in the LP will affect ESs that local residents depend on (Fu et al., 2013). Linking ecological processes to ESs can help understand the complex relationships among ESs and support optimized ecosystem management measures (e.g. minimize trade-offs and maximize synergies) and sustainability of CHANSs (Fu et al., 2013). Regarding this aspect, a large number of studies about land use and land cover change, ES assessments, and their trade-off and synergy analysis have been conducted in the LP over the past two decades.
Ecological restoration has changed the LP's land use and land cover (Fig. 3). From 2000 to 2015, cultivated land in the LP decreased by 12.06 %, while shrubland, grassland, artificial surfaces, and water bodies increased by 19.64 %, 3.76 %, 96.02 %, and 20.21 %, respectively (Liu et al., 2019). The long-term AVHRR (Advanced Very High Resolution Radiometer) NDVI average changed after 1999, the slope increased from 0.0018 yr−1 in the 1982–1998 period to 0.0053 yr−1 in 1999–2015 (Wu et al., 2019). The piecewise linear regression demonstrated that most breakpoints in the NDVI time series were detected between 1999 and 2002 (Wu et al., 2019). This means that the LP has been greening since the GTGP implementation in 1999.
By combining multi-source data, such as land use and land cover, remote sensing, and statistics, and by utilizing site-based monitoring data to set localized parameters, many ES assessments have been conducted in the LP at multiple scales (Lu et al., 2014; Zheng et al., 2016; Su et al., 2020). Using the universal soil loss equation (USLE, ), the soil conservation service was quantified, and the results showed that total soil erosion in the LP significantly decreased by 0.96×108 t yr−1 between 2000 and 2015 (Wu et al., 2019). The sediment load between the Toudaotai and Tongguan stations decreased significantly by 0.25×108 t yr−1 during the same period (Wu et al., 2019). Accordingly, the nutrient (N and P) retention service increased in the areas subjected to revegetation (Li et al., 2019). The carbon sequestration service, which was quantified by the terrestrial Carnegie–Ames–Stanford approach (CASA) ecosystem model, showed a significant increasing trend. Estimates showed that the total regional NPP increased by 9.3±1.3 between 2000 and 2010 (Feng et al., 2016). The spatial extent of the increased NPP and ET observed from MODIS coincides with that of GTGP implementation (Feng et al., 2016), indicating that vegetation restoration, rather than climate change, is the main cause of the estimated increase of annual NPP or ET in the LP. However, water-related ESs, such as water supply and baseflow regulation, decreased dramatically at watershed (Luo et al., 2019) and regional scales (Lü et al., 2012; Wang et al., 2017). In contrast, the food provision service did not seem to be negatively affected by the reduction in cultivated land (Lü et al., 2012; Li et al., 2019), and the total grain output in the LP increased by 56.7 % from 2000 to 2015 due to improved agricultural production techniques (Wu et al., 2019).
The assessments of ESs further provide the basis for the analysis of their trade-off and synergy relationships (Fig. 3). Overall, the same ES types (e.g. provisioning and regulating services) tended to show synergistic relationships, possibly due to the fact that they shared similar ecosystem processes and drivers (Bennett et al., 2009). For example, four provisioning services in the LP, namely grain production, oil crop production, livestock supply, and water supply, showed synergistic relationships, the exception being that between water supply and oil crop production (Li et al., 2019), and three regulating services, namely, carbon sequestration, soil conservation, and N and P retention, were positively correlated with each other (Li et al., 2019). Although the negative impact of vegetation restoration on agricultural production is small, the trade-off between food supply and regulating services still exists in some local areas. The trade-off between water yield and soil conservation and the trade-off between water yield and carbon sequestration services have received more attention because of the importance of water resources for the sustainability of the LP ecosystem (Jia et al., 2014; Su et al., 2020). In addition, there are scale effects on the trade-offs and synergies among ESs. For example, studies have reported that NPP and water yield and sediment retention and water yield showed trade-offs at the watershed scale, while they were not correlated at the LP scale (Su et al., 2020). Therefore, it is necessary to elucidate the relationships among ESs at multiple scales to promote their sustainable use and facilitate policymaking. Hu et al. (2015) developed a spatial assessment and optimization tool for regional ESs (SAORES) to evaluate and optimize regional water yield, soil erosion control, carbon sequestration, and food supply services in the LP. Based on multi-objective trade-offs among ESs, SAORES was able to provide suggestions for maximizing key ESs and optimizing land use patterns in the context of the GTGP (Hu et al., 2015).
In summary, the assessments of critical ESs and the analyses of their trade-off and synergy relationships in the LP provide theoretical support for the spatial optimization of ESs. However, there are still research gaps that should be considered in future investigations. First, current studies mainly focused on the provision of ES, but the ES demands and well-being of local residents have been largely neglected. The residents of the LP are stakeholders with an interest in ESs and ecosystem management, and neglecting their demand and well-being may threaten the sustainability of ecological restoration outcomes (Dong et al., 2021). Second, as distant regions are connected by telecoupling processes, the use of ESs in one region may be affected by the management of ESs in other locations (Koellner et al., 2019); however, current studies mainly focus on place-based assessments of ESs, largely neglecting the flow of ESs between regions.
4.3 Social–ecological sustainability
Studies that coupled patterns and processes and linked processes to services can reveal the interactions among social–ecological components and help us figure out the causes of the unsustainable status of a CHANS and the corresponding solutions. Although the vegetation coverage has improved greatly and soil erosion has been well controlled in the LP after 2 decades of ecological restoration, some accompanied issues have started to threaten the long-term sustainability of the LP. The carbon–water conflict accompanied with revegetation is a typical example (Feng et al., 2016). Due to high-density planting, introduction of exotic plant species, and mismanagement of planted vegetation, large areas subjected to revegetation suffered soil drying (Chen et al., 2015; Wang et al., 2011). Studies have shown that the current vegetation cover has already exceeded the climate-defined equilibrium vegetation cover in many parts of the LP (Zhang et al., 2018). Considering the minimum water needed for socioeconomic activities, local revegetation in the plateau is approaching sustainable water resource limits (Feng et al., 2016), threatening the sustainability of the CHANS. In addition, although the total grain production in the LP increased because of the development of agricultural facilities and application of modern technologies (Wu et al., 2019), some counties still faced a decrease in grain production due to the conversion of cropland to forest or grassland, and further revegetation may threaten the local food supply (Chen et al., 2015). To obtain a higher yield from the declining cropland areas, local people tended to increase the use of agrochemicals to maintain or enhance land productivity (Wang et al., 2014). As a result, diffused pollution from agriculture has increased, and the quality of local land, groundwater, and surface water have been affected (Wang et al., 2014). Another important issue is the spillover effects of the LP on the telecoupled regions. In the past, the expansion of cultivation and deforestation to meet local food demand caused environmental degradation and severe soil erosion in the LP (Fu et al., 2017). The sediments produced flowed into the Yellow River, leading to the rising of riverbed levels (Chen et al., 2015) and extension of the Yellow River delta (Kong et al., 2015). After ecological restoration, the improved environment and decreased soil erosion in the LP led to the subsequent reduction of sediment load and runoff in the lower Yellow River (Wu et al., 2020). The Yellow River delta has shifted to an erosional phase (Bi et al., 2014), which might affect more than 2 million people and the biodiversity in distant but coupled ecosystems (Zhou et al., 2015). Based on a deep understanding of the ecohydrological effects caused by vegetation restoration, changes in critical ESs and their trade-off and synergy relationships, the social–ecological sustainability in the LP can be enhanced through the following targeted policies and management (Fig. 3).
-
Enhancing water and food security. The LP is mainly located in arid and semi-arid regions, and water security is the priority issue that should be considered by scientists and policymakers (Lu et al., 2014). In response to this issue, some overplanted areas may need to be reasonably thinned to reduce vegetation density, and a better fit of plant species and planting density to the LP's natural environment (e.g. precipitation gradient), as well as adaptive management of revegetation areas, are needed for the ensuing ecological restoration process (Lü et al., 2012; Feng et al., 2016). Considering that new large-scale vegetation restoration has deviated from the current social–ecological contexts of the LP, future restoration should be more refined and targeted, pay more attention to the key ecologically vulnerable areas, and avoid the one-size-fits-all governance approaches (Wang et al., 2017). Besides, it is necessary to consider the impact of climate change on NPP and water yield to meet future challenges of water shortage. To achieve food security, relevant stakeholders should better manage the existing croplands, adopt advanced agricultural production and water-saving techniques to improve food yield per unit area, and simultaneously avoid agricultural pollution. In addition, more new croplands can be created by filling gullies, where conditions allow it (Q. Liu et al., 2013; Wang et al., 2018a).
-
Implementing basin-wide management. Scientists and policymakers need to consider not only the local ecohydrological effects caused by vegetation restoration in the LP but also its spillover effects on distant regions through telecoupling processes (Wu et al., 2020). The establishment of a basin-wide ecosystem and land use management regime is needed to support sustainable water use and sediment regulation (Zhou et al., 2015; Wang et al., 2016, 2017). The interconnected sub-hydrological units of the Yellow River basin span various human-defined boundaries and are managed by different agents, resulting in institutional fragmentation. Considering the holistic nature of the basin, policymakers and managers of the LP should cooperate and coordinate with middle-stream and downstream stakeholders to integrate management of river water and sediment (Wang et al., 2019). Specifically, cross-border and cross-scale coordination exerted by a higher-level authority is an effective means to overcome institutional fragmentation. This is because a third party or a higher administrative agency with whole-basin responsibility can promote effective coordination on a basin level by establishing social ties indirectly linking actors across administrative levels (Wang et al., 2019).
-
Maintaining the GTGP achievements. The sustainability of the conservation achievements obtained from the GTGP is affected by the post-programme land use plans of participants (Page and Bellotti, 2015; Deng et al., 2016). A study of the effects of regional ESs changes and individual characteristics on participants' post-programme land reconversion willingness in a watershed of the LP has shown that participants with a higher household income, more household employment change, higher household involvement in crop production, and higher ecological awareness tended to not reconvert their GTGP land to agriculture use (Wu et al., 2021b). At the regional level, the changes in water yield and grain production services would affect the reconversion willingness of participants with different individual characteristics (Wu et al., 2021b). With a more comprehensive understanding of the effects of multiple-level factors on the reconversion willingness, the sustainability of the GTGP achievements can be enhanced by diversifying and improving the household income of participants (Bryan et al., 2018), enhancing the participants' ecological awareness and recognition of ecological benefits obtained from the programme (Song et al., 2014), and providing place-based solutions and protection priorities of ESs (Wu et al., 2021b).
-
Promoting rural livelihood transition. The overdependence of the local people on agriculture for livelihood is the main cause of the previous social–ecological trap in the LP (Wu et al., 2021b). Promoting rural livelihood transition toward a development path with low ecological impact is critical for the long-term social–ecological sustainability of the LP. The GTGP converted numerous croplands to forest or grassland, which released local rural labour from crop production, promoted the transformation to non-farm activities (Liu et al., 2008; Uchida et al., 2009), improved rural household income, and simultaneously decreased income inequality (Li et al., 2011). In parallel to the GTGP, multiple factors like economic development, industrialization, and urbanization also influence the livelihoods and income of rural households (Ouyang et al., 2016; Bryan et al., 2018). A comprehensive analysis proved that the local economy and investment played dominant roles in the improvement of rural household income in the LP, while tourism, investment, and rural migration could boost non-farm work participation and effectively use the surplus labourers released from the GTGP, indirectly increasing income (Wu et al., 2021a). To promote socioeconomic development in the LP and enhance the benefits that participants gain from non-farm work, it is necessary to promote initiatives that can create more local non-farm employment and help non-farm participation, such as promoting the tourism industry, attracting more visitors and investment from outside, and providing training for new earning skills (Wu et al., 2021a; Cao, 2011; Yin et al., 2014). Meanwhile, barriers for rural labour migration should be overcome by offering equal opportunities and information services to migrant workers (Yang et al., 2018), especially to rural labourers that have fewer local employment opportunities and wages.
In this review, the LP was used as an example to illustrate the adoption of the pattern–process–service–sustainability paradigm to analyse the dynamics of CHANS and identify management priorities to enhance sustainability. After the implementation of the GTGP in 1999, the LP has achieved the general “win–win” gains of restoring the environment and promoting socioeconomic development (Wu et al., 2019). However, water limits and land shortages are becoming the boundary of regional development (Wang et al., 2018a). It is necessary to readjust the revegetation strategy and promote socioeconomic development and livelihood transition and consequently the social–ecological sustainability of the LP (Wang et al., 2018a; Li et al., 2017). By revealing the limitations of current studies, future research using the pattern–process–service–sustainability paradigm to observe, analyse, and understand the dynamics and changes of CHANSs should especially focus on the following aspects.
-
Integrated research on multiple processes. CHANSs are complex systems in which multiple natural and social processes interact and co-evolve (Quintas-Soriano et al., 2021), and therefore only focusing on one or a few of them may not reveal the essential laws behind complex phenomena (Fu et al., 2019). Multiscale systematic research quantifying the interactions among the hydrological, pedologic, atmospheric, biological, and socioeconomic processes is necessary to provide integrative and convincing guidance to achieve sustainability (Fu, 2020). The driving mechanisms behind these interactions should also be further studied. This proposed research can specifically investigate the interactions among water, soil, atmosphere, and ecosystem, and their ecological effects; the biogeochemical processes and mechanisms on the Earth's surface; and the interactions of different earth spheres and their response mechanisms to global change (Fu, 2020).
-
Cascades of ecosystem structure, function, services, and human well-being. As a link between nature and human society, the ES concept has become an important component of the scientific discourse, and a research object in science and policy (Carpenter et al., 2009). Understanding the ES delivery process from ecosystems to human society is essential to effectively manage ecosystems (Mandle et al., 2021). The research frontiers of ES cascades involve the identification of the spatial mismatch between ES supply and demand, the differentiation of ES supply and human use, and the analysis of interregional ES flows to support cross-regional management cooperation (Koellner et al., 2019; Vallecillo et al., 2019; Zwierzchowska et al., 2018). In addition, the relationship between ESs and human well-being has also become an important research topic (Mandle et al., 2021). Further research needs to explore the coupling mechanisms of ES cascade components and clarify the dominant mechanisms of ES trade-offs to enhance and optimize ESs for the achievement of regional ecological security.
-
Feedback mechanisms of human and natural systems. Human and natural systems interact and co-evolve over time and have substantial impacts upon each other, with causality operating in both directions (Fu and Li, 2016; Quintas-Soriano et al., 2021). Understanding the complex interactions and feedbacks of CHANSs is one of the core research objectives in the field of sustainability. Future research needs to examine the bidirectional feedbacks between human and natural systems, the dynamics and resilience of CHANSs, the interactions between humans and nature at multiple scales (e.g. telecoupling), the structural and dynamic fit of human and natural systems, and the capacity boundaries of CHANSs (Wang et al., 2018b).
-
Data and models for sustainability. Field monitoring, control experiments, and remote sensing of natural systems at multiple scales provide abundant datasets to understand ecological processes (Wei et al., 2010; Feng et al., 2010; Yao et al., 2012; Zhou et al., 2016; Liang et al., 2018), while the development of information technology and big data can help detect human activities and strengthen the spatiotemporal links between socioeconomic and natural processes. However, due to the scale mismatch between natural and socioeconomic processes, data assimilation is needed to integrate data from different scales, disciplines, and sources and form a CHANS dataset for further sustainability analyses (Fu, 2020). In addition to the system dataset, the development of a model, or model systems, is crucial for integrated CHANS research. This kind of model can be realized through the integration of multiple ecological, human, and socioeconomic models (Fu and Li, 2016).
A deep understanding of the reciprocal effect between landscape patterns and ecological processes and the complex linkages between ecological processes and ESs that support human well-being is crucial for promoting social–ecological sustainability. The conceptual cascade framework of pattern–process–service–sustainability proposed in this review can help researchers observe, analyse, and understand the dynamics of CHANSs, diagnose the causes of the unsustainable status, and support the design of policies and measures that promote sustainability. To illustrate the use of this framework, this review systematically examines the CHANS research experience in China's LP, in terms of coupling landscape patterns and ecological processes, linking ecological processes to ESs, and promoting social–ecological sustainability.
Since 2000, the vegetation coverage in the LP has increased and soil erosion has been well controlled due to ecological restoration. However, overplanting, the introduction of exotic plant species, and the mismanagement of planted vegetation have also led to soil drying in some regions subjected to revegetation, and a trade-off between carbon sequestration and water supply has been identified at multiple scales. Some social–ecological issues, such as water resource limits, local food scarcity, and negative spillover effects, have emerged in the LP, posing a threat to its sustainable development in the future. To promote social–ecological sustainability, scientists and policymakers should pay more attention to water and food security, basin-wide governance, maintenance of ecological restoration achievements, and rural livelihood transition.
Considering the typicality of the social–ecological context in the LP, we believe that research experience from this region are relevant for CHANS studies in the rest of the world. Future research using the pattern–process–service–sustainability paradigm should especially examine the integrated research on multiple processes; the cascades of ecosystem structure, function, services, and human well-being; the feedback mechanisms of human and natural systems; and the data and models for sustainability.
No datasets were used in this article.
BF designed the research. XuW, ZW, and XiW performed the literature review. BF, XuW, ZW, SW, and XiW contributed to the interpretation and writing.
The contact author has declared that neither they nor their co-authors have any competing interests.
Publisher's note: Copernicus Publications remains neutral with regard to jurisdictional claims in published maps and institutional affiliations.
This article was presented in the EGU General Assembly 2021 in session – “EGU 2020/2021 Alexander von Humboldt Medal Lectures”, 23 April 2021.
This research has been supported by the National Natural Science Foundation of China (grant no. 41930649), the Chinese Academy of Sciences (grant no. QYZDY-SSW-DQC025), the China National Postdoctoral Program for Innovative Talents (grant no. BX2021042), and the China Postdoctoral Science Foundation (grant no. 2021M700458).
This paper was edited by Ning Zeng and reviewed by three anonymous referees.
An, W., Li, Z., Wang, S., Wu, X., Lu, Y., Liu, G., and Fu, B.: Exploring the effects of the “Grain for Green” program on the differences in soil water in the semi-arid Loess Plateau of China, Ecol. Eng., 107, 144–151, https://doi.org/10.1016/j.ecoleng.2017.07.017, 2017.
Barton, L., Newsome, S. D., Chen, F. H., Wang, H., Guilderson, T. P., and Bettinger, R. L.: Agricultural origins and the isotopic identity of domestication in northern China, P. Natl. Acad. Sci. USA, 106, 5523–5528, https://doi.org/10.1073/pnas.0809960106, 2009.
Bennett, E. M., Peterson, G. D., and Gordon, L. J.: Understanding relationships among multiple ecosystem services, Ecol. Lett., 12, 1394–1404, https://doi.org/10.1111/j.1461-0248.2009.01387.x, 2009.
Bi, N., Wang, H., and Yang, Z.: Recent changes in the erosion–accretion patterns of the active Huanghe (Yellow River) delta lobe caused by human activities, Cont. Shelf Res., 90, 70–78, 2014.
Biggs, R., de Vos, A., Preiser, R., Clements, H., Maciejewski, K., and Schlüter, M.: The Routledge Handbook of Research Methods for Social-Ecological Systems, Routledge, https://doi.org/10.4324/9781003021339, 2021.
Bryan, B. A., Gao, L., Ye, Y., Sun, X., Connor, J. D., Crossman, N. D., Stafford-Smith, M., Wu, J., He, C., Yu, D., Liu, Z., Li, A., Huang, Q., Ren, H., Deng, X., Zheng, H., Niu, J., Han, G., and Hou, X.: China's response to a national land-system sustainability emergency, Nature, 559, 193–204, https://doi.org/10.1038/s41586-018-0280-2, 2018.
Cao, S.: Impact of China's Large-Scale Ecological Restoration Program on the Environment and Society in Arid and Semiarid Areas of China: Achievements, Problems, Synthesis, and Applications, Crit. Rev. Env. Sci. Tec., 41, 317–335, https://doi.org/10.1080/10643380902800034, 2011.
Carpenter, S. R., Mooney, H. A., Agard, J., Capistrano, D., Defries, R. S., Diaz, S., Dietz, T., Duraiappah, A. K., Oteng-Yeboah, A., Pereira, H. M., Perrings, C., Reid, W. V., Sarukhan, J., Scholes, R. J., and Whyte, A.: Science for managing ecosystem services: Beyond the Millennium Ecosystem Assessment, P. Natl. Acad. Sci. USA, 106, 1305–1312, https://doi.org/10.1073/pnas.0808772106, 2009.
Chen, L., Wei, W., Fu, B., and Lü, Y.: Soil and water conservation on the Loess Plateau in China: Review and perspective, Prog. Phys. Geog., 31, 389–403, https://doi.org/10.1177/0309133307081290, 2007.
Chen, L., Wang, J., Wei, W., Fu, B., and Wu, D.: Effects of landscape restoration on soil water storage and water use in the Loess Plateau Region, China, Forest Ecol. Manag., 259, 1291–1298, https://doi.org/10.1016/j.foreco.2009.10.025, 2010.
Chen, Y.: Flood dynamics of the lower Yellow River over the last 3000 years: Characteristics and implications for geoarchaeology, Quatern. Int., 521, 147–157, https://doi.org/10.1016/j.quaint.2019.05.040, 2019.
Chen, Y., Syvitski, J. P. M., Gao, S., Overeem, I., and Kettner, A. J.: Socio-economic Impacts on Flooding: A 4000-Year History of the Yellow River, China, Ambio, 41, 682–698, https://doi.org/10.1007/s13280-012-0290-5, 2012.
Chen, Y., Wang, K., Lin, Y., Shi, W., Song, Y., and He, X.: Balancing green and grain trade, Nat. Geosci., 8, 739–741, https://doi.org/10.1038/ngeo2544, 2015.
Costanza, R., De Groot, R., Braat, L., Kubiszewski, I., Fioramonti, L., Sutton, P., Farber, S., and Grasso, M.: Twenty years of ecosystem services: How far have we come and how far do we still need to go?, Ecosyst. Serv., 28, 1–16, https://doi.org/10.1016/j.ecoser.2017.09.008, 2017.
Cumming, G.: Theoretical Frameworks for the Analysis of Social-Ecological Systems, in: Social-ecological systems in transition, edited by: Sakai, S. and Umetsu, C., Springer, Tokyo, 3–24, https://doi.org/10.1007/978-4-431-54910-9_1, 2014.
Deng, J., Sun, P., Zhao, F., Han, X., Yang, G., and Feng, Y.: Analysis of the ecological conservation behavior of farmers in payment for ecosystem service programs in eco-environmentally fragile areas using social psychology models, Sci. Total Environ., 550, 382–390, https://doi.org/10.1016/j.scitotenv.2016.01.152, 2016.
Dong, X., Wang, X., Wei, H., Fu, B., Wang, J., and Uriarte-Ruiz, M.: Trade-offs between local farmers' demand for ecosystem services and ecological restoration of the Loess Plateau, China, Ecosyst. Serv., 49, 101295, https://doi.org/10.1016/j.ecoser.2021.101295, 2021.
Feng, X., Fu, B., Yang, X., and Lü, Y.: Remote sensing of ecosystem services: An opportunity for spatially explicit assessment, Chinese Geogr. Sci., 20, 522–535, https://doi.org/10.1007/s11769-010-0428-y, 2010.
Feng, X., Fu, B., Lu, N., Zeng, Y., and Wu, B.: How ecological restoration alters ecosystem services: An analysis of carbon sequestration in China's Loess Plateau, Sci. Rep.-UK, 3, 2846, https://doi.org/10.1038/srep02846, 2013.
Feng, X., Fu, B., Piao, S., Wang, S., Ciais, P., Zeng, Z., Lü, Y., Zeng, Y., Li, Y., Jiang, X., and Wu, B.: Revegetation in China's Loess Plateau is approaching sustainable water resource limits, Nat. Clim. Change, 6, 1019–1022, https://doi.org/10.1038/nclimate3092, 2016.
Fu, B.: Promoting Geography for Sustainability, Geography and Sustainability, 1, 1–7, https://doi.org/10.1016/j.geosus.2020.02.003, 2020.
Fu, B. and Li, Y.: Bidirectional coupling between the Earth and human systems is essential for modeling sustainability, Natl. Sci. Rev., 3, 397–398, https://doi.org/10.1093/nsr/nww094, 2016.
Fu, B. and Wei, Y.: Editorial overview: Keeping fit in the dynamics of coupled natural and human systems, Curr. Opin. Env. Sust., 33, A1–A4, https://doi.org/10.1016/j.cosust.2018.07.003, 2018.
Fu, B., Liang, D., and Lu, N.: Landscape ecology: Coupling of pattern, process, and scale, Chinese Geogr. Sci., 21, 385, https://doi.org/10.1007/s11769-011-0480-2, 2011a.
Fu, B., Liu, Y., Lü, Y., He, C., Zeng, Y., and Wu, B.: Assessing the soil erosion control service of ecosystems change in the Loess Plateau of China, Ecol. Complex., 8, 284–293, https://doi.org/10.1016/j.ecocom.2011.07.003, 2011b.
Fu, B., Wang, S., Su, C., and Forsius, M.: Linking ecosystem processes and ecosystem services, Curr. Opin. Env. Sust., 5, 4–10, https://doi.org/10.1016/j.cosust.2012.12.002, 2013.
Fu, B., Zhang, L., Xu, Z., Zhao, Y., Wei, Y., and Skinner, D.: Ecosystem services in changing land use, J. Soil. Sediment., 15, 833–843, https://doi.org/10.1007/s11368-015-1082-x, 2015.
Fu, B., Shuai, W., Yu, L., Jianbo, L., Wei, L., and Chiyuan, M.: Hydrogeomorphic Ecosystem Responses to Natural and Anthropogenic Changes in the Loess Plateau of China, Annu. Rev. Earth Pl. Sc., 45, 223–243, https://doi.org/10.1146/annurev-earth-063016-020552, 2017.
Fu, B., Tian, T., Liu, Y., and Zhao, W.: New Developments and Perspectives in Physical Geography in China, Chinese Geogr. Sci., 29, 363–371, https://doi.org/10.1007/s11769-019-1038-y, 2019.
Gao, G., Ma, Y., and Fu, B.: Multi-temporal scale changes of streamflow and sediment load in a loess hilly watershed of China, Hydrol. Process., 30, 365–382, https://doi.org/10.1002/hyp.10585, 2016.
Gao, G., Zhang, J., Liu, Y., Ning, Z., Fu, B., and Sivapalan, M.: Spatio-temporal patterns of the effects of precipitation variability and land use/cover changes on long-term changes in sediment yield in the Loess Plateau, China, Hydrol. Earth Syst. Sci., 21, 4363–4378, https://doi.org/10.5194/hess-21-4363-2017, 2017.
Gunderson, L., Cosens, B. A., Chaffin, B. C., Arnold, C. A., Fremier, A. K., Garmestani, A. S., Craig, R. K., Gosnell, H., Birge, H. E., Allen, C. R., Benson, M. H., Morrison, R. R., Stone, M. C., Hamm, J. A., Nemec, K., Schlager, E., and Llewellyn, D.: Regime shifts and panarchies in regional scale social-ecological water systems, Ecol. Soc., 22, 31, https://doi.org/10.5751/es-08879-220131, 2017.
He, G., Wang, Z., Shen, J., Cui, Z., and Zhang, F.: Transition of agriculture on the Loess Plateau of China toward green development, Front. Agr. Sci. Eng., 8, 491–500, https://doi.org/10.15302/j-fase-2021428, 2021.
Hu, H., Fu, B., Lü, Y., and Zheng, Z.: SAORES: a spatially explicit assessment and optimization tool for regional ecosystem services, Landscape Ecol., 30, 547–560, https://doi.org/10.1007/s10980-014-0126-8, 2015.
Intergovernmental Science-Policy Platform on Biodiversity and Ecosystem Services: Summary for policymakers of the global assessment report on biodiversity and ecosystem services (summary for policy makers), IPBES Plenary at its seventh session (IPBES 7, Paris, 2019), Zenodo, https://doi.org/10.5281/zenodo.3553579, 2019.
Jia, X., Fu, B., Feng, X., Hou, G., Liu, Y., and Wang, X.: The tradeoff and synergy between ecosystem services in the Grain-for-Green areas in Northern Shaanxi, China, Ecol. Indic., 43, 103–113, https://doi.org/10.1016/j.ecolind.2014.02.028, 2014.
Koellner, T., Bonn, A., Arnhold, S., Bagstad, K. J., Fridman, D., Guerra, C. A., Kastner, T., Kissinger, M., Kleemann, J., Kuhlicke, C., Liu, J., López-Hoffman, L., Marques, A., Martín-López, B., Schulp, C. J. E., Wolff, S., and Schröter, M.: Guidance for assessing interregional ecosystem service flows, Ecol. Indic., 105, 92–106, https://doi.org/10.1016/j.ecolind.2019.04.046, 2019.
Kong, D., Miao, C., Borthwick, A. G. L., Duan, Q., Liu, H., Sun, Q., Ye, A., Di, Z., and Gong, W.: Evolution of the Yellow River Delta and its relationship with runoff and sediment load from 1983 to 2011, J. Hydrol., 520, 157–167, https://doi.org/10.1016/j.jhydrol.2014.09.038, 2015.
Lewis, S. L. and Maslin, M. A.: Defining the Anthropocene, Nature, 519, 171–180, https://doi.org/10.1038/nature14258, 2015.
Li, J., Feldman, M. W., Li, S., and Daily, G. C.: Rural household income and inequality under the Sloping Land Conversion Program in western China, P. Natl. Acad. Sci. USA, 108, 7721–7726, https://doi.org/10.1073/pnas.1101018108, 2011.
Li, T., Lu, Y., Fu, B., Comber, A. J., Harris, P., and Wu, L.: Gauging policy-driven large-scale vegetation restoration programmes under a changing environment: Their effectiveness and socio-economic relationships, Sci. Total Environ., 607–608, 911–919, https://doi.org/10.1016/j.scitotenv.2017.07.044, 2017.
Li, T., Lü, Y., Fu, B., Hu, W., and Comber, A. J.: Bundling ecosystem services for detecting their interactions driven by large-scale vegetation restoration: enhanced services while depressed synergies, Ecol. Indic., 99, 332–342, https://doi.org/10.1016/j.ecolind.2018.12.041, 2019.
Liang, H., Xue, Y., Li, Z., Wang, S., Wu, X., Gao, G., Liu, G., and Fu, B.: Soil moisture decline following the plantation of Robinia pseudoacacia forests: Evidence from the Loess Plateau, Forest Ecol. Manag., 412, 62–69, https://doi.org/10.1016/j.foreco.2018.01.041, 2018.
Liu, J., Dietz, T., Carpenter, S. R., Alberti, M., Folke, C., Moran, E., Pell, A. N., Deadman, P., Kratz, T., Lubchenco, J., Ostrom, E., Ouyang, Z., Provencher, W., Redman, C. L., Schneider, S. H., and Taylor, W. W.: Complexity of Coupled Human and Natural Systems, Science, 317, 1513–1516, https://doi.org/10.1126/science.1144004, 2007.
Liu, J., Li, S., Ouyang, Z., Tam, C., and Chen, X.: Ecological and socioeconomic effects of China's policies for ecosystem services, P. Natl. Acad. Sci. USA, 105, 9477–9482, 2008.
Liu, J., Dietz, T., Carpenter, S. R., Folke, C., and Trade, S. W. T. M.: Coupled Human and Natural Systems, Ambio, 36, 639–649, 2009.
Liu, J., Hull, V., Batistella, M., Defries, R., Dietz, T., Fu, F., Hertel, T. W., Izaurralde, R. C., Lambin, E. F., Li, S., Martinelli, L. A., McConnell, W. J., Moran, E. F., Naylor, R., Ouyang, Z., Polenske, K. R., Reenberg, A., De Miranda Rocha, G., Simmons, C. S., Verburg, P. H., Vitousek, P. M., Zhang, F., and Zhu, C.: Framing Sustainability in a Telecoupled World, Ecol. Soc., 18, 26, https://doi.org/10.5751/es-05873-180226, 2013.
Liu, J., Dietz, T., Carpenter, S. R., Taylor, W. W., Alberti, M., Deadman, P., Redman, C., Pell, A., Folke, C., Ouyang, Z., and Lubchenco, J.: Coupled human and natural systems: The evolution and applications of an integrated framework, Ambio, 50, 1778–1783, https://doi.org/10.1007/s13280-020-01488-5, 2021.
Liu, Q., Wang, Y., Zhang, J., and Chen, Y.: Filling gullies to create farmland on the loess plateau, Environ. Sci. Technol., 47, 7589–7590, https://doi.org/10.1021/es402460r, 2013.
Liu, Y., Fu, B., Lü, Y., Wang, Z., and Gao, G.: Hydrological responses and soil erosion potential of abandoned cropland in the Loess Plateau, China, Geomorphology, 138, 404–414, https://doi.org/10.1016/j.geomorph.2011.10.009, 2012.
Liu, Y., Lü, Y., Fu, B., Harris, P., and Wu, L.: Quantifying the spatio-temporal drivers of planned vegetation restoration on ecosystem services at a regional scale, Sci. Total Environ., 650, 1029–1040, https://doi.org/10.1016/j.scitotenv.2018.09.082, 2019.
Lu, N., Fu, B., Jin, T., and Chang, R.: Trade-off analyses of multiple ecosystem services by plantations along a precipitation gradient across Loess Plateau landscapes, Landscape Ecol., 29, 1697–1708, https://doi.org/10.1007/s10980-014-0101-4, 2014.
Lü, Y., Fu, B., Feng, X., Zeng, Y., Liu, Y., Chang, R., Sun, G., and Wu, B.: A Policy-Driven Large Scale Ecological Restoration: Quantifying Ecosystem Services Changes in the Loess Plateau of China, PLoS One, 7, e31782, https://doi.org/10.1371/journal.pone.0031782, 2012.
Luo, Y., Lü, Y., Fu, B., Zhang, Q., Li, T., Hu, W., and Comber, A.: Half century change of interactions among ecosystem services driven by ecological restoration: Quantification and policy implications at a watershed scale in the Chinese Loess Plateau, Sci. Total Environ., 651, 2546–2557, https://doi.org/10.1016/j.scitotenv.2018.10.116, 2019.
MA: Ecosystems and Human Well-being: Synthesis, Island Press, Washington, DC, ISBN 1-59726-040-1, 2005.
Mandle, L., Shields-Estrada, A., Chaplin-Kramer, R., Mitchell, M. G. E., Bremer, L. L., Gourevitch, J. D., Hawthorne, P., Johnson, J. A., Robinson, B. E., Smith, J. R., Sonter, L. J., Verutes, G. M., Vogl, A. L., Daily, G. C., and Ricketts, T. H.: Increasing decision relevance of ecosystem service science, Nature Sustainability, 4, 161–169, https://doi.org/10.1038/s41893-020-00625-y, 2021.
Nelson, D. R., Adger, W. N., and Brown, K.: Adaptation to Environmental Change: Contributions of a Resilience Framework, Annu. Rev. Environ. Resour., 32, 395–419, https://doi.org/10.1146/annurev.energy.32.051807.090348, 2007.
Ostrom, E.: A General Framework for Analyzing Sustainability of Social-Ecological Systems, Science, 325, 419–422, https://doi.org/10.1126/science.1172133, 2009.
Ouyang, Z., Zheng, H., Xiao, Y., Polasky, S., Liu, J., Xu, W., Wang, Q., Zhang, L., Xiao, Y., Rao, E., Jiang, L., Lu, F., Wang, X., Yang, G., Gong, S., Wu, B., Zeng, Y., Yang, W., and Daily, G. C.: Improvements in ecosystem services from investments in natural capital, Science, 352, 1455–1459, https://doi.org/10.1126/science.aaf2295, 2016.
Page, G. and Bellotti, B.: Farmers value on-farm ecosystem services as important, but what are the impediments to participation in PES schemes?, Sci. Total Environ., 515–516, 12–19, https://doi.org/10.1016/j.scitotenv.2015.02.029, 2015.
Partelow, S.: A review of the social-ecological systems framework: applications, methods, modifications, and challenges, Ecol. Soc., 23, 36, https://doi.org/10.5751/es-10594-230436, 2018.
Quintas-Soriano, C., Brandt, J., Baxter, C. V., Bennett, E. M., Requena-Mullor, J. M., and Castro, A. J.: A framework for assessing coupling and de-coupling trajectories in river social-ecological systems, Sustain. Sci., 17, 121–134, https://doi.org/10.1007/s11625-021-01048-0, 2021.
Rocha, J. C., Peterson, G. D., and Biggs, R.: Regime Shifts in the Anthropocene: Drivers, Risks, and Resilience, PLoS One, 10, e0134639, https://doi.org/10.1371/journal.pone.0134639, 2015.
Song, C., Zhang, Y., Mei, Y., Liu, H., Zhang, Z., Zhang, Q., Zha, T., Zhang, K., Huang, C., and Xu, X.: Sustainability of forests created by China's sloping land conversion program: a comparison among three sites in Anhui, Hubei and Shanxi, Forest Policy Econ., 38, 161–167, 2014.
Steffen, W., Crutzen, P. J., and Mcneill, J. R.: The Anthropocene: are humans now overwhelming the great forces of Nature?, Ambio, 36, 614–621, 2007.
Steffen, W., Broadgate, W., Deutsch, L., Gaffney, O., and Ludwig, C.: The trajectory of the Anthropocene: The Great Acceleration, The Anthropocene Review, 2, 81–98, 2015.
Su, C., Dong, M., Fu, B., and Liu, G.: Scale effects of sediment retention, water yield, and net primary production: A case-study of the Chinese Loess Plateau, Land Degrad. Dev., 31, 1408–1421, https://doi.org/10.1002/ldr.3536, 2020.
Sun, Z., Shao, J., Liu, L., Cui, J., Bonomo, M. F., Guo, Q., Wu, X., and Wang, J.: The first Neolithic urban center on China's north Loess Plateau: The rise and fall of Shimao, Archaeological Research in Asia, 14, 33–45, https://doi.org/10.1016/j.ara.2017.02.004, 2018.
Tian, Q.: Complex Adaptive Systems and a Sustainability Framework, in: Rural Sustainability, Springer, Cham, 1–14, https://doi.org/10.1007/978-3-319-52685-0_1, 2017.
Uchida, E., Rozelle, S., and Xu, J.: Conservation payments, liquidity constraints, and off-farm labor: impact of the Grain-for-Green Program on rural households in China, Am. J. Agr. Econ., 91, 70–86, 2009.
UN: Transforming our world: the 2030 Agenda for sustainable development, UN, New York, https://digitallibrary.un.org/record/3923923/files/A_RES_70_1-EN.pdf (last access: 11 March 2022), 2015.
UN: The Sustainable Development Goals Report 2019, United Nations, New York, ISBN 9789211014037, 2019.
Vallecillo, S., La Notte, A., Ferrini, S., and Maes, J.: How ecosystem services are changing: an accounting application at the EU level, Ecosyst. Serv., 40, 101044, https://doi.org/10.1016/j.ecoser.2019.101044, 2019.
Wang, S., Fu, B., Gao, G., Liu, Y., and Zhou, J.: Responses of soil moisture in different land cover types to rainfall events in a re-vegetation catchment area of the Loess Plateau, China, Catena, 101, 122–128, https://doi.org/10.1016/j.catena.2012.10.006, 2013.
Wang, S., Fu, B., Piao, S., Lü, Y., Ciais, P., Feng, X., and Wang, Y.: Reduced sediment transport in the Yellow River due to anthropogenic changes, Nat. Geosci., 9, 38–41, https://doi.org/10.1038/ngeo2602, 2016.
Wang, S., Fu, B., Liang, W., Liu, Y., and Wang, Y.: Driving forces of changes in the water and sediment relationship in the Yellow River, Sci. Total Environ., 576, 453–461, https://doi.org/10.1016/j.scitotenv.2016.10.124, 2017.
Wang, S., Fu, B., Chen, H., and Liu, Y.: Regional development boundary of China's Loess Plateau: Water limit and land shortage, Land Use Policy, 74, 130–136, https://doi.org/10.1016/j.landusepol.2017.03.003, 2018a.
Wang, S., Fu, B., Zhao, W., Liu, Y., and Wei, F.: Structure, function, and dynamic mechanisms of coupled human–natural systems, Curr. Opin. Env. Sust., 33, 87–91, https://doi.org/10.1016/j.cosust.2018.05.002, 2018b.
Wang, S., Fu, B., Bodin, Ö., Liu, J., Zhang, M., and Li, X.: Alignment of social and ecological structures increased the ability of river management, Sci. Bull., 64, 1318–1324, https://doi.org/10.1016/j.scib.2019.07.016, 2019.
Wang, T., Wu, J., Kou, X., Oliver, C., Mou, P., and Ge, J.: Ecologically asynchronous agricultural practice erodes sustainability of the Loess Plateau of China, Ecol. Appl., 20, 1126–1135, https://doi.org/10.1890/09-0229.1, 2010.
Wang, X., Shen, J., and Zhang, W.: Emergy evaluation of agricultural sustainability of Northwest China before and after the grain-for-green policy, Energ. Policy, 67, 508–516, https://doi.org/10.1016/j.enpol.2013.12.060, 2014.
Wang, Y., Shao, M., Zhu, Y., and Liu, Z.: Impacts of land use and plant characteristics on dried soil layers in different climatic regions on the Loess Plateau of China, Agr. Forest Meteorol., 151, 437–448, https://doi.org/10.1016/j.agrformet.2010.11.016, 2011.
Wei, W., Chen, L., Fu, B., Huang, Z., Wu, D., and Gui, L.: The effect of land uses and rainfall regimes on runoff and soil erosion in the semi-arid loess hilly area, China, J. Hydrol., 335, 247–258, https://doi.org/10.1016/j.jhydrol.2006.11.016, 2007.
Wei, W., Chen, L., Fu, B., and Chen, J.: Water erosion response to rainfall and land use in different drought-level years in a loess hilly area of China, Catena, 81, 24–31, https://doi.org/10.1016/j.catena.2010.01.002, 2010.
Wu, X., Wang, S., Fu, B., Feng, X., and Chen, Y.: Socio-ecological changes on the Loess Plateau of China after Grain to Green Program, Sci. Total Environ., 678, 565–573, https://doi.org/10.1016/j.scitotenv.2019.05.022, 2019.
Wu, X., Wei, Y., Fu, B., Wang, S., Zhao, Y., and Moran, E. F.: Evolution and effects of the social-ecological system over a millennium in China's Loess Plateau, Sci. Adv., 6, eabc0276, https://doi.org/10.1126/sciadv.abc0276, 2020.
Wu, X., Liu, J., Fu, B., Wang, S., and Wei, Y.: Integrating multiple influencing factors in evaluating the socioeconomic effects of payments for ecosystem services, Ecosyst. Serv., 51, 101348, https://doi.org/10.1016/j.ecoser.2021.101348, 2021a.
Wu, X., Wang, S., and Fu, B.: Multilevel analysis of factors affecting participants' land reconversion willingness after the Grain for Green Program, Ambio, 50, 1394–1403, https://doi.org/10.1007/s13280-020-01475-w, 2021b.
Yang, H., Yang, W., Zhang, J., Connor, T., and Liu, J.: Revealing pathways from payments for ecosystem services to socioeconomic outcomes, Sci. Adv., 4, eaao6652, https://doi.org/10.1126/sciadv.aao6652, 2018.
Yao, X., Fu, B., Lü, Y., Chang, R., Wang, S., Wang, Y., and Su, C.: The multi-scale spatial variance of soil moisture in the semi-arid Loess Plateau of China, J. Soil. Sediment., 12, 694–703, https://doi.org/10.1007/s11368-012-0481-5, 2012.
Yin, R., Liu, C., Zhao, M., Yao, S., and Liu, H.: The implementation and impacts of China's largest payment for ecosystem services program as revealed by longitudinal household data, Land Use Policy, 40, 45–55, https://doi.org/10.1016/j.landusepol.2014.03.002, 2014.
Yu, Y., Zhao, W., Martinez-Murillo, J. F., and Pereira, P.: Loess Plateau: from degradation to restoration, Sci. Total Environ., 738, 140206, https://doi.org/10.1016/j.scitotenv.2020.140206, 2020.
Zhang, S., Yang, D., Yang, Y., Piao, S., Yang, H., Lei, H., and Fu, B.: Excessive Afforestation and Soil Drying on China's Loess Plateau, J. Geophys. Res.-Biogeo., 123, 923–935, https://doi.org/10.1002/2017JG004038, 2018.
Zhao, G., Mu, X., Wen, Z., Wang, F., and Gao, P.: Soil erosion, conservation, and eco-environment changes in the Loess Plateau of China, Land Degrad. Dev., 24, 499–510, https://doi.org/10.1002/ldr.2246, 2013.
Zheng, Z., Fu, B., and Feng, X.: GIS-based analysis for hotspot identification of tradeoff between ecosystem services: A case study in Yanhe Basin, China, Chinese Geogr. Sci., 26, 466–477, https://doi.org/10.1007/s11769-016-0816-z, 2016.
Zhou, J., Fu, B., Gao, G., Lü, Y., Liu, Y., Lü, N., and Wang, S.: Effects of precipitation and restoration vegetation on soil erosion in a semi-arid environment in the Loess Plateau, China, Catena, 137, 1–11, https://doi.org/10.1016/j.catena.2015.08.015, 2016.
Zhou, Y., Huang, H. Q., Nanson, G. C., Huang, C., and Liu, G.: Progradation of the Yellow (Huanghe) River delta in response to the implementation of a basin-scale water regulation program, Geomorphology, 243, 65–74, 2015.
Zhu, H., Fu, B., Wang, S., Zhu, L., Zhang, L., Jiao, L., and Wang, C.: Reducing soil erosion by improving community functional diversity in semi-arid grasslands, J. Appl. Ecol., 52, 1063–1072, https://doi.org/10.1111/1365-2664.12442, 2015.
Zwierzchowska, I., Hof, A., Iojă, I.-C., Mueller, C., Poniży, L., Breuste, J., and Mizgajski, A.: Multi-scale assessment of cultural ecosystem services of parks in Central European cities, Urban For. Urban Gree., 30, 84–97, https://doi.org/10.1016/j.ufug.2017.12.017, 2018.
- Abstract
- Introduction
- The pattern–process–service–sustainability framework
- China's Loess Plateau
- Application of the framework in the LP
- Future directions of the pattern–process–service–sustainability paradigm
- Conclusions
- Data availability
- Author contributions
- Competing interests
- Disclaimer
- Acknowledgements
- Financial support
- Review statement
- References
pattern–process–service–sustainabilitycascade framework. The use of this framework is systematically illustrated by a review of CHANS research experience in China's Loess Plateau in terms of coupling landscape patterns and ecological processes, linking ecological processes to ecosystem services, and promoting social–ecological sustainability.
- Abstract
- Introduction
- The pattern–process–service–sustainability framework
- China's Loess Plateau
- Application of the framework in the LP
- Future directions of the pattern–process–service–sustainability paradigm
- Conclusions
- Data availability
- Author contributions
- Competing interests
- Disclaimer
- Acknowledgements
- Financial support
- Review statement
- References