the Creative Commons Attribution 4.0 License.
the Creative Commons Attribution 4.0 License.
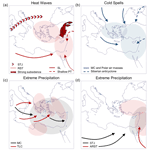
Extreme weather and societal impacts in the eastern Mediterranean
Assaf Hochman
Francesco Marra
Gabriele Messori
Joaquim G. Pinto
Shira Raveh-Rubin
Yizhak Yosef
Georgios Zittis
Gaining a holistic understanding of extreme weather, from its physical drivers to its impacts on society and ecosystems, is key to supporting future risk reduction and preparedness measures. Here, we provide an overview of the state of the art, knowledge gaps and key open questions in the study of extreme weather events over the vulnerable eastern Mediterranean. This region is situated in a transition zone between subtropical and mid-latitude climates. The large-scale atmospheric circulation and its interaction with regional synoptic systems (i.e., Cyprus Lows, Red Sea Troughs, Persian Troughs, “Sharav” Lows) and high-pressure systems mainly govern extreme weather. Complex orographic features further play an important role in the generation of extreme weather. Most extreme weather events, including heavy precipitation, cold spells, floods and windstorms, are associated with Cyprus Lows or active Red Sea Troughs, whereas heat waves are related with either Persian Troughs and sub-tropical high-pressure systems in summer or the Sharav Low during springtime. In future decades, heat waves and droughts are projected to significantly increase in both frequency and intensity. Changes in heavy precipitation may vary in sign and magnitude depending on the scale, severity and region of interest. There are still relatively large uncertainties concerning the physical understanding and the projected changes of cold spells, windstorms and compound extremes, as these types of events received comparatively little attention in the literature. We further identify knowledge gaps that relate to the societal impacts of extreme weather. These gaps mainly relate to the effects extreme weather may have on mortality, morbidity and infrastructure in the eastern Mediterranean. Research is currently limited in this context, and we recommend strengthening the database of analyzed case studies. We trust that this can only be suitably accomplished by inter-disciplinary and international regional collaboration (in spite of political unrest).
- Article
(10388 KB) - Full-text XML
-
Supplement
(837 KB) - BibTeX
- EndNote
- Included in Encyclopedia of Geosciences
Weather and climate extremes such as heat waves, cold spells, heavy precipitation, droughts, windstorms and compound extremes have detrimental socio-economic and ecological impacts. These include excess mortality (e.g., Ryti et al., 2016; Ballester et al., 2019; Charlton-Perez et al., 2019), agricultural losses (e.g., Deryng et al., 2014; Ferrarezi et al., 2019) and ecosystem damage (e.g., Williams, 2014; Caldeira et al., 2015; Boucek et al., 2016). For this reason, a better understanding of extreme events has been identified as one of the World Climate Research Program's Grand Challenges (https://www.wcrp-climate.org/gc-extreme-events, last access: 29 March 2022). A plethora of studies indicate that extreme weather events may intensify and/or become more frequent with climate change, making this topic all the more relevant (e.g., Rummukainen, 2012; Samuels et al., 2018; Hochman et al., 2018a; Perkins-Kirkpatrick and Lewis, 2020).
The Mediterranean region has been identified as a climate change “hotspot” (Giorgi, 2006; Barcikowska et al., 2020) located in a transition zone between mid-latitude and sub-tropical climates. The eastern Mediterranean is characterized by persistent hot and dry weather conditions during summer and changeable temperatures and rainy spells during winter (Kushnir et al., 2017). The region is influenced by mid-latitude, subtropical and tropical weather systems (Alpert et al., 2005), which can lead to a range of extremes, including windstorms, hydrological extremes and temperature extremes. Although the eastern Mediterranean is most commonly affected by heat waves and drought, it has also experienced several flood events and cold spells in recent decades.
Current regional climate projections indicate that the eastern Mediterranean may become warmer and drier in the future (e.g., Hochman et al., 2018c; Zittis et al., 2019; Cherif et al., 2020). Moreover, a tendency towards more frequent weather extremes has also been projected (e.g., Samuels et al., 2018). From an environmental point of view, these kinds of extremes are often associated with enhanced flood (e.g., Tarolli et al., 2012; Zoccatelli et al., 2019) and drought potential (e.g., Cook et al., 2016).
In the last 2 decades, there has been a continuous increase in the number of studies on extreme weather events and their possible impacts on society and ecosystems in the eastern Mediterranean. The region has also been the focus of a number of review papers dealing with climate variability (Finnè et al., 2011; Lelieveld et al., 2012), climate change impacts on health (Khader et al., 2015) and atmospheric conditions conducive to heavy precipitation (Dayan et al., 2015). However, there has not yet been a review with a broad focus on extreme weather events and a synthesis of the potential future research directions to pursue.
The main objective of this paper is to review the state of the art on extreme weather research in the eastern Mediterranean, with a specific focus on the physical understanding, observed trends and future projections. Moreover, we also aim to identify the key societal impacts associated with such extreme weather events under current and future climate conditions.
The paper is organized as follows: Sects. 2–5 summarize the current knowledge on temperature extremes (heat waves and cold spells), hydrological extremes (heavy precipitation and drought), wind extremes and compound extremes, respectively. Each section is partitioned into subsections focusing on physical understanding, observed trends, future projections and the main societal impacts. Section 6 summarizes the main points and knowledge gaps. Section 7 presents our recommendations for future research in the form of overarching key open questions.
2.1 Physical understanding
Heat waves are commonly defined as prolonged periods of exceptionally hot weather. Although heat waves are one of the most common natural hazards, there is still no universally accepted definition for a heat wave. While some local authorities and researchers use fixed temperature thresholds for a number of consecutive days, others apply percentile-based definitions to account for local climatic peculiarities and gain a uniform perspective on observed changes in climate extremes. For instance, the widely used Warm Spell Duration Index (WSDI) is defined as the annual number of days belonging to events of at least 6 consecutive days with a maximum temperature (TX) exceeding the local 90th percentile derived from a given base period (e.g., 1961–1990). This index, along with the Cold Spell Duration Index (annual number of days belonging to events of at least 6 consecutive days with minimum temperature, TN, below the 10th percentile), was defined by the Expert Team on Climate Change Detection and Indices (Klein Tank et al., 2009; Zhang et al., 2011).
Both the occurrence and intensity of heat waves over the eastern Mediterranean are mostly related to large-scale atmospheric circulation patterns and flow anomalies. The latter can induce strong subsidence, adiabatic warming, warm-air advection and positive radiation anomalies as a result of clear skies (Fig. 1a; Palecki et al., 2001; Black et al., 2004; Fink et al., 2004; Lipton et al., 2005; Baldi et al., 2006; Maheras et al., 2006). Brikas et al. (2006) provide evidence that intense heat waves over Greece primarily occur when an anticyclonic subtropical jet is situated to the northwest of the Balkans (Fig. 1a). In addition, low soil moisture may amplify extreme heat waves in the region (Fink et al., 2004; Fischer et al., 2007; Jaeger and Seneviratne, 2011; Mueller and Seneviratne, 2012; Zittis et al., 2014). During summer heat waves, a relatively shallow Persian Trough is associated with weakened Etesian winds from eastern Europe and the Mediterranean into the Levant (the region along the eastern Mediterranean coasts), implying a reduction in the prevailing cool advection from the northwest (Figs. 1a and 2e, f; Saaroni et al., 2003). This causes a lowering of the marine inversion base height, and consequently the temperatures rise mainly in mountainous regions. Relative humidity increases on the coastal plain and lowlands, leading to intense heat stress and warm nights there (Yosef et al., 2019). During spring, “Sharav” Low episodes may induce extremely hot temperatures over the region (Fig. 2g, h). The associated cyclone tracks typically tend to closely follow the northern African coastline along with an upper-level trough far to the west (Fig. 1a). These cyclones move eastward relatively quickly, accompanied by high temperatures, low humidity and strong winds that may lead to heavy dust storms (Alpert and Ziv, 1989). Another synoptic system inducing eastern Mediterranean heat waves is the Red Sea Trough. When the trough's axis is to the west of 35∘ E, it is associated with warm and dry conditions resulting from easterly or southeasterly flow from the desert at near-surface levels over the southeastern part of the region (Fig. 1a; Tsvieli and Zangvil, 2007; Saaroni et al., 2020; Hochman et al., 2021b).
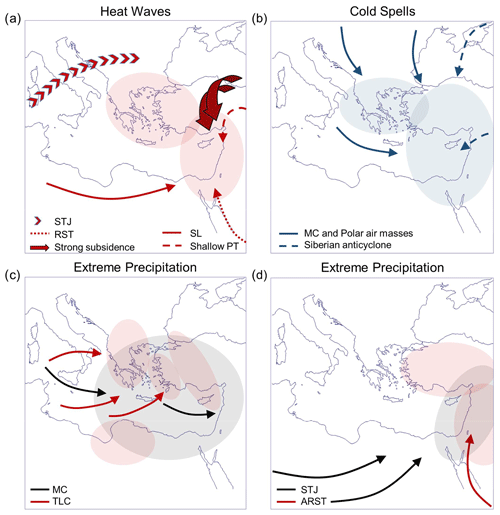
Figure 1A schematic of the main air mass origins (arrows) and impacted areas (ellipses) of synoptic and large-scale systems bringing heat waves during spring, autumn, and summer (a); cold spells during winter (b); and heavy precipitation during winter (c) and autumn (d) to the eastern Mediterranean. (a) Heat waves can be attributed to the following phenomena: Sharav Lows (SL – solid line), Red Sea Troughs or Arabian Troughs (RST – dotted line), shallow Persian Troughs (PT – dashed line), strong subsidence (two thick arrows), or an anomalous subtropical jet (STJ – chevrons). (b) Cold spells can be attributed to the following phenomena: polar air masses and Mediterranean Cyclones or Cyprus Lows (MC – solid lines) and Siberian anticyclone (dashed lines). Heavy precipitation can be attributed to the following phenomena: (c) during winter, Mediterranean cyclones or Cyprus Lows (MC – solid black lines) and tropical-like cyclones (TLC – solid red lines); (d) during autumn to an anomalous subtropical jet (STJ – solid black lines) or active Red Sea Trough (ARST – solid red line).
While the eastern Mediterranean is typically associated with warm weather and heat waves, it also experiences winter cold spells. Polar air mass outbreaks, originating from continental regions north of the eastern Mediterranean in Asia and Europe, lead to unique weather conditions characterized by cold, dry and stable weather (Fig. 1b; Saaroni et al., 1996). North–northeasterly flow over the eastern Mediterranean is derived from an omega-shaped anticyclone over eastern Europe and a trough originating in the Arabian Peninsula extending towards the easternmost margin of the basin. This introduces flow anomalies that favor cold spells over the region (Fig. 1b; Kostopoulou and Jones, 2007). Pappas et al. (2004) provide further evidence that cold spells in Greece can also occur when a persistent northwestern Siberian anticyclone reaches its highest intensity during winter (Fig. 1b). In addition, extratropical low-pressure systems forming over the Mediterranean Sea, often termed Cyprus Lows (e.g., Alpert and Shay-El, 1994), associated with a pronounced upper-level trough and a more eastern surface cyclone center, can drive severe cold spells accompanied by snowfall over high altitudes of the Levant (Fig. 2a, b; Hochman et al., 2020d).
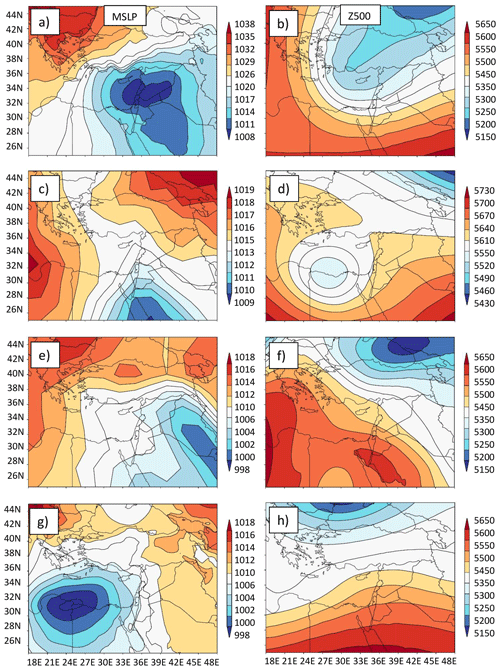
Figure 2Examples for extreme configurations of the main synoptic systems over the eastern Mediterranean. Mean sea level pressure (MSLP, in hPa; left column) and 500 hPa geopotential height (Z500, in m; right column) based on ERA5 daily reanalysis data (Hersbach et al., 2020) are shown. A Cyprus Low associated with heavy precipitation and strong winds over large parts of the eastern Mediterranean on 11 December 2013 is shown in (a, b). A Red Sea Trough associated with deadly flash floods in the Dead Sea on 24 April 2018 is shown in (c, d). A Persian Trough associated with a record-breaking heat wave in large parts of the Middle East on 15 August 2010 is shown in (e, f). A Sharav Low associated with an extreme heat wave over parts of the eastern Mediterranean on 15 March 1998 is shown in (g, h).
2.2 Observed trends and future projections
A general warming has been observed over the Mediterranean in the 20th century (Alpert et al., 2008; Tanarhte et al., 2012; Mariotti et al., 2015). For example, significant annually and seasonally increasing trends were detected in the frequency of percentile-based indices of warm days (TX90p, i.e., days when TX > 90th percentile) and nights (TN90p, i.e., days when TN > 90th percentile) over Israel, showing stronger trends in the last 30 years relative to the long-term period (e.g., 1950–2020; Yosef et al., 2019). In contrast, the cold indices (TX and TN below the 10th percentile of the same long-term period) showed a significant decrease in the number of cold days and nights. The most significant temperature trends were revealed in summer, where both maximum and minimum temperature extremes exhibited statistically significant warming trends. Such warm/cold extreme trends were reported in several studies over the eastern Mediterranean (e.g., Kostopoulou and Jones, 2005; Zhang et al., 2005; Kioutsioukis et al., 2010; Donat et al., 2014; Yosef et al., 2019). In addition, heat wave duration has been significantly increasing (Kostopoulou and Jones, 2005; Zhang et al., 2005; Kuglitsch et al., 2010; Yosef et al., 2019), whereas cold spell durations showed only weakly decreasing trends (Zhang et al., 2005; Yosef et al., 2019).
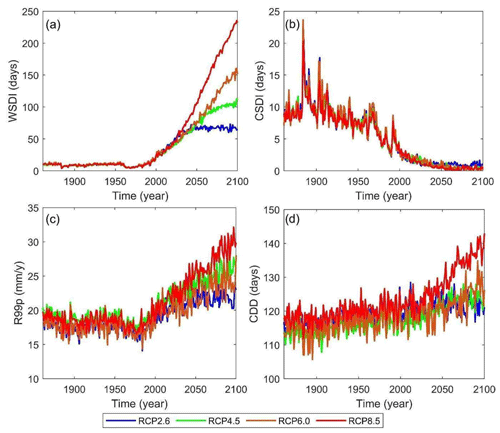
Figure 3Annual CMIP5 ensemble mean projections of extreme indices over the eastern Mediterranean (Taylor et al., 2012; 25–45∘ N, 18–48∘ E; see Fig. 2 for the domain and Supplement Table S1 for list of models used). (a) Warm Spell Duration Index (WSDI, in d). (b) Cold Spell Duration Index (CSDI, in d). (c) Annual total precipitation when daily precipitation exceeds the 99th percentile of wet-day precipitation (R99p in mm yr−1). (d) Maximum number of consecutive days per year with less than 1 mm of precipitation (CDD, in d). The reference period is 1981–2010. The following four representative concentration pathways are considered: RCP2.6 (blue), RCP4.5 (green), RCP6.0 (brown) and RCP8.5 (red).
Based on climate models' projections, the current increasing trend of heat wave frequency, duration and intensity is expected to continue or even accelerate in the future (Fig. 3a; e.g., Zittis et al., 2016). In general, the eastern Mediterranean and the Middle East may be exposed to increased heat wave intensities, with the average peak temperature of the hottest yearly event increasing by 6–10 ∘C, mainly due to the overall mean warming and stronger summer anticyclone conditions by the end of the century (Zittis et al., 2016). Recently, Zittis et al. (2021a) showed that under a business-as-usual pathway (RCP8.5) “severe”, “extreme” and “very extreme” heat waves would likely become the norm by 2050–2070, while the so far unprecedented “super-extreme” and “ultra-extreme” events are expected to prevail by the end of the century. Moreover, the occurrence of very hot days (TX > 35 ∘C) and tropical nights (TN > 25 ∘C), as well as the heat stress (a combination of threshold-based indices of hot days and tropical nights), are expected to increase by 1 to 3 months by the end of the century (Kostopoulou et al., 2014; Zittis et al., 2016). Due to the overall mean warming, heat wave peak temperature will be higher, along with an increase in other extreme temperature indices (e.g., TX90p, TN90p), as reflected in regional climate simulations (Lelieveld et al., 2016; Zittis et al., 2016; Hochman et al., 2018c). The frequency of cold spells is likely to decrease, yet their severity and duration does not show significant changes (Kodra et al., 2011). Specifically, in the eastern Mediterranean and Middle East, the cold spell duration index is projected to decrease by about 3–5 d (RCP4.5 and RCP8.5) relative to the base period 1981–2000 by the end of the 21st century (Fig. 3b; Sillmann et al., 2013).
Since many heat wave and cold spell indices are percentile based, it should be noted here that these indices are extremely sensitive to the selected reference period, especially in a world characterized by continuous warming. As such, the increasing trend in the magnitude of heat waves is reduced when a warmer and more recent reference period, e.g., 1981–2010, is used, rather than a “colder” one, e.g., 1961–1990 (Yosef et al., 2021).
2.3 Societal impacts
The current increasing trends in heat wave frequency and intensity, as well as the accelerated projected changes, will affect many sectors of society. For the health sector, heat waves can lead to increased hospital admissions and excess human morbidity and mortality, particularly among the elderly and infirm (Naumann et al., 2020). A clear relationship between high temperatures and cardiovascular mortality by cerebrovascular, ischemic and other heart diseases has been found (Basu and Samet, 2002; Gosling et al., 2009; Lubczyńska et al., 2015). Agriculture and water supply systems are also vulnerable to heat waves through damage to crops and vegetation and higher water demand (e.g., Papadaskalopoulou et al., 2020). Pests and diseases could also present a serious threat. In addition, frost days may also be a considerable risk to crops and vegetation in the region (Cramer et al., 2018). An increased energy consumption, e.g., greater demand for air conditioning in homes and offices, infrastructure stress and even shifts in touristic preferences due to the higher temperatures are also expected (Nairn and Fawcett, 2013; Naumann et al., 2020).
These adverse effects might be amplified by increasing urbanization (Grimm et al., 2008; United Nations, Department of Economic and Social Affairs, Population Division, 2019), transported or local air pollution (Solberg et al., 2008; Tressol et al., 2008), and the urban heat island effect (Mandelmilch et al., 2020). Specifically, Cramer et al. (2018) noted that the combination of the aforementioned impacts in the Mediterranean basin may exacerbate their magnitude or could produce successive and more frequent stress periods that the least resilient countries would find difficult to cope with.
3.1 Heavy precipitation
3.1.1 Physical understanding
Heavy precipitation events in the eastern Mediterranean are related to a variety of synoptic systems with distinct dynamics, moisture sources, precipitation yields, intensities and scales (Fig. 1c, d; Alpert et al., 2004; Dayan et al., 2015; Armon et al., 2018). The frequency and intensity of heavy precipitation events display large regional and local variations (Kostopoulou and Jones, 2005; Nastos and Zerefos, 2009) related to the dominant synoptic systems and to their interaction with local conditions (Nastos et al., 2013b; de Vries et al., 2018; Armon et al., 2020).
Many of the heavy precipitation events are associated with Cyprus Lows peaking in winter (Figs. 1c, 2a, b; Alpert and Shay-El, 1994; Almazroui et al., 2015; Ziv et al., 2015; Lionello et al., 2016; Kushnir et al., 2017; Armon et al., 2018; Mastrantonas et al., 2021). Mediterranean cyclones often embed convective and stratiform processes with numerous precipitation cells (Peleg and Morin, 2012; Belachsen et al., 2017; Flaounas et al., 2022). Their precipitation efficiency, defined as the ratio between precipitation yield and moisture influx (Sui et al., 2007), is moderate (Armon et al., 2018), but convective cells may deliver extreme intensities over small scales (Belachsen et al., 2017; Armon et al., 2018). The large availability of moisture supply from the Mediterranean Sea may result in persistent extremes over large areas (Alpert et al., 2004; Flaounas et al., 2015; Raveh-Rubin and Wernli, 2015; Armon et al., 2018).
On rare occasions, intense mesoscale vortices with dynamics similar to tropical cyclones influence the western parts of the region (Fig. 1c; Zhang et al., 2021). These are commonly referred to as Mediterranean tropical-like cyclones or “medicanes”, i.e., Mediterranean hurricanes (Miglietta, 2019). Medicanes develop in a way analogous to tropical cyclones, via wind-induced surface heat exchange (Emanuel, 1986; Miglietta and Rotunno, 2019). However, some recent studies have reported on cases with different development mechanisms (Mazza et al., 2017; Fita and Flaounas, 2018). Precipitation patterns resemble the ones of Mediterranean cyclones, albeit on smaller spatial scales (Flaounas et al., 2018a, b; Zhang et al., 2021). In some areas, medicanes contribute up to 2 %–5 % of extreme precipitation days (Zhang et al., 2021) and often have dramatic impacts even though they are very rare (∼ one every 2 years; Nastos et al., 2018).
Except for the Mediterranean Sea, other sources of moisture can also lead to heavy precipitation in the eastern Mediterranean. The Red Sea Trough is a stationary surface trough extending from the African Monsoon low over equatorial Africa toward the eastern Mediterranean. When associated with an amplified Rossby wave, this system is often termed an active Red Sea Trough. Under these conditions, it transfers abundant moisture from the Arabian and Red seas to the eastern Mediterranean, leading to local torrential rains mostly during autumn (Figs. 1d and 2c, d; Krichak et al., 1997a; de Vries et al., 2013; Armon et al., 2018; Baseer et al., 2019). Precipitation typically occurs in the form of numerous localized thunderstorms and mesoscale convective systems (Krichak et al., 1997b; Dayan et al., 2001; Belachsen et al., 2017; Marra and Morin, 2018). Despite their relatively low occurrence frequency (< 5 % of the rainy days; Tsvieli and Zangvil, 2005; Awad and Almazroui, 2016) and low precipitation efficiency (Armon et al., 2018), active Red Sea Troughs are responsible for ∼ 38 % of the flash floods in the semi-arid and arid regions of the Levant (Kahana et al., 2002; Dayan and Morin, 2006).
Subtropical jet disturbances may occasionally bring moisture from equatorial regions or other sources to the eastern Mediterranean, in so-called “tropical plumes” (Fig. 1d; Rubin et al., 2007; Tubi and Dayan, 2014; Armon et al., 2018). Although they rarely reach the region (as only a few events are historically documented), subtropical jet disturbances are characterized by high precipitation efficiency over regional scales, with widespread intense rainfall that may last for several days (Dayan and Abramski, 1983; Armon et al., 2018) and lead to significant impacts especially during autumn (Dayan and Morin, 2006).
3.1.2 Observed trends and future projections
Paleo-climatic evidence suggests the existence of important links between mean climatic conditions and occurrence and intensity of the different synoptic systems inducing heavy precipitation in the region (Enzel et al., 2003; Benito et al., 2015; Ahlborn et al., 2018; Armon et al., 2018; Ben Dor et al., 2018; Armon et al., 2019; Morin et al., 2019; Lu et al., 2020; Ludwig and Hochman, 2022). Our understanding of ongoing and future changes in heavy precipitation events thus stems from our ability to detect and predict changes in the occurrence and intensity of all these systems (Toreti et al., 2010; Ziv et al., 2014; Merkenschlager et al., 2017; Marra et al., 2019b).
Statistically significant trends in extreme precipitation have indeed been reported in recent years (Alpert et al., 2002; Nastos and Zerefos, 2008; Yosef et al., 2009; Mathbout et al., 2018; Ajjur and Riffi, 2020), whose sign and level of significance depend on the studied area. Interestingly, some of these trends were not found to be significant about a decade ago (Zhang et al., 2005; Shohami et al., 2011; Ziv et al., 2014), suggesting that either the records were not long enough to robustly identify statistically significant trends (Morin, 2011) or that the trend has accelerated. Additionally, a complicated dependence of the fine-scale spatiotemporal structure of convective cells on temperature has been reported (Peleg et al., 2018b), implying that climate change might also affect these characteristics.
Climate models consistently project a substantial decrease in the number of Mediterranean cyclones reaching the southeastern portion of the Mediterranean basin (up to 35 % decrease by the end of the 21st century under the RCP8.5 scenario), along with a decrease in their mean daily precipitation yield (Pinto et al., 2007; Kelley et al., 2012; Zappa et al., 2015a; Hochman et al., 2018a, b, 2020b; Samuels et al., 2018; Reale et al., 2021). A slight increase in the occurrence frequency of Red Sea Troughs has been reported, accompanied by a decrease of their typical intensities (Zappa et al., 2015a; Hochman et al., 2018a, 2021b; Saaroni et al., 2020), although there is still a debate on whether the number of active Red Sea Troughs is actually decreasing (Hochman et al., 2021b). A decrease in the occurrence frequency of medicanes is also estimated, together with some evidence for an increase in their intensity (Cavicchia et al., 2014; Romera et al., 2016; Tous et al., 2016; González-Alemán et al., 2019), with yet to be studied impacts on the emerging extremes (e.g., Hosseini et al., 2020). To the best of our knowledge, climate change impact studies on subtropical jet disturbances are not yet available. Overall, the sign and significance of changes in heavy precipitation events are still unclear due to the large uncertainties inherent in the analysis of extreme events (Fatichi et al., 2016; Peleg et al., 2018a; Zittis et al., 2021b). Recent findings suggest the sign of change might depend on the event severity, with increasing trends for larger extremes and decreasing trends for smaller, but still rare, intensities. The rarest extremes may thus increase in intensity in spite of the decrease in both occurrence and typical intensity of Mediterranean cyclones and the decreased intensity of active Red Sea Troughs (Fig. 3c; Marra et al., 2021a; Zittis et al., 2021b). Pseudo-global-warming convection-permitting models show a rather complicated response of heavy precipitation to climate change, with decreased storm wet area (−40 %) and decreased storm rainfall amounts (−30 %) in spite of increased rain rates (+15 %) and extreme rain rates (+22 %, Armon et al., 2022). The analysis of synoptic systems in climate models, via detection and tracking algorithms (e.g., Neu et al., 2013; Lionello et al., 2016; Hochman et al., 2019a; Saaroni et al., 2020), could help in understanding their future response to climate change, with important implications for the quantification of heavy precipitation event frequency.
3.1.3 Societal impacts
Heavy precipitation events are crucial for the water resources of the region (Samuels et al., 2009; Peleg et al., 2012, 2015a, b; Levy et al., 2020; Givati et al., 2019). These events also constitute the main trigger of floods and landslides (Kahana et al., 2002; Ocakoglu et al., 2002; David-Novak et al., 2004) and force major erosive and geomorphic effects (Abdallah, 2012; Michaelides et al., 2018; Armon et al., 2019; Shmilovitz et al., 2020).
The impact of local and regional heavy precipitation events depends largely on precipitation yield, local peak intensities and spatiotemporal scales (Kahana et al., 2002). Accurate representation of their patterns and location is thus crucial for understanding and predicting local impacts (Morin and Yakir, 2014; Yucel and Onen, 2014; Zoccatelli et al., 2019; Armon et al., 2020; Rinat et al., 2021). The convective component makes heavy precipitation events in the region effective in delivering large amounts of rainfall over small areas and short periods, causing pluvial and urban flooding (Diakakis et al., 2017) and flash floods (Dayan and Morin, 2006; Llasat et al., 2010; Farhan and Anbar, 2014). In fact, flash floods represent one of the most damaging meteorological hazards in the whole Mediterranean (Barredo 2007; Llasat et al., 2010; Tarolli et al., 2012; Petrucci et al., 2019). Occasionally, heavy precipitation events are responsible for landslide movements and triggering of debris flow (Ocakoglu et al., 2002; David-Novak et al., 2004) and for major erosive and geomorphic responses (Inbar et al., 1998; Grodek et al., 2012; Avni et al., 2016; Shmilovitz et al., 2020, 2021).
Documenting the local impact of heavy precipitation requires high-resolution observations from weather radars, satellites and cellular links to complement in-situ measurements (Messer et al., 2006; Morin et al., 2007; Koutroulis and Tsanis, 2010; Miglietta et al., 2013; Amponsah et al., 2018; Rinat et al., 2018; Borga et al., 2019; Diakakis et al., 2019; Varlas et al., 2019; Laviola et al., 2020; Rinat et al., 2021). This is due to the small spatiotemporal scales of precipitation patterns and their effects on the ground. Atmospheric indices can sometimes provide valuable information at the regional scale (Morsy et al., 2020), but effective forecasting of local impacts is still challenging due to the small scales and short response times of the basins (Collier, 2007; Morin et al., 2009; Borga et al., 2014; Zoccatelli et al., 2020). Recent developments in convection-permitting modeling and nowcasting techniques may lead to improvements (e.g., Coppola et al., 2020), but forecasting the location of small-scale extreme occurrences remains elusive, and proper forecasting should adopt probabilistic ensemble approaches (Toros et al., 2018; Armon et al., 2020; Spyrou et al., 2020; Rinat et al., 2021).
Risk assessment generally relies on precipitation frequency analysis and intensity–duration–frequency curves (Koutsoyiannis et al., 1998; Koutsoyiannis and Baloutsos, 2000; Ben-Zvi, 2009; Fathy et al., 2020; Marra et al., 2020; Nastos et al., 2021), while envelope curves are often used to identify regional upper limits for flood peak discharge (Tarolli et al., 2012; Amponsah et al., 2020). However, the coastal, orographic and climatic structure of the region, together with the typically small scales of high-impact events and the relatively scarce availability of long observational records, make extreme frequency analysis challenging (Peleg et al., 2018b; Diakakis et al., 2020; Metzger et al., 2020). The presence of heavy precipitation associated with different synoptic systems and characterized by different scales, intensities and interactions with local features, makes the quantification of risk even more challenging. As such, novel statistical techniques may help in isolating and quantifying trends (Miniussi and Marani, 2020), as well as in understanding the underlying mechanisms (Marra et al., 2019b). Additionally, they could leverage the distributed information from remotely sensed data sets to improve our understanding of the impact of local conditions on the development and statistics of extremes (Marra et al., 2019a, 2021b).
3.2 Droughts
3.2.1 Physical understanding
Droughts are periods of abnormally dry conditions that are long enough to cause a serious hydrological imbalance (e.g., Wilhite and Glantz, 1985). They can occur at any time or any place in the world and are considered natural disasters. They are generally classified into four categories: (i) meteorological, (ii) agricultural, (iii) hydrological and (iv) socio-economic droughts (Wilhite and Glantz, 1985). A more recent definition also includes ecological droughts (Crausbay et al., 2017). The timescales of interest depend on the impact under investigation, and usually range from weekly to multiannual. Here, we primarily focus on meteorological droughts, which are very relevant for the eastern Mediterranean that frequently experiences prolonged dry weather periods. The eastern Mediterranean is located at subtropical latitudes, in which high-pressure systems suppress cloud formation and precipitation, particularly during summer. Exceptions can occur in high-elevation areas in the northern parts of the eastern Mediterranean (mainly in southern Balkans and Anatolia), where orography and/or convective activity can also trigger precipitation during summer (Funatsu et al., 2009). Prolonged dry periods in the eastern Mediterranean can also occur in other seasons, but these are mainly driven by internal climate variability and large-scale modes or teleconnections that can suppress cyclogenesis within the Mediterranean or can shift storm tracks to northern latitudes (Sousa et al., 2011). Spring and summer droughts in the Middle East have been associated with negative phases of the North Atlantic Oscillation (Vicente-Serrano et al., 2011), while an opposite correlation is found over parts of Turkey and Greece for winter, spring and summer droughts (Sousa et al., 2011; Vicente-Serrano et al., 2011). The East Atlantic Pattern shows a similar spatial footprint to the North Atlantic Oscillation, albeit only for winter droughts, with positive correlations over the western part of the eastern Mediterranean. Remote sea surface temperature anomalies, primarily over the Atlantic, may also show a teleconnection with compound drought occurrences in this region (Sousa et al., 2011).
While lack of precipitation is the main driver of drought, other meteorological variables, such as abnormally high temperatures, radiation, evapotranspiration or low soil moisture can augment drought severity. Besides, due to the growth of population and expansion of agricultural, energy and industrial sectors, water demand has increased manifold and water scarcity has been occurring almost every year in many parts of the world, including in the eastern Mediterranean (Mishra and Singh, 2010). Several metrics for assessing drought frequency and severity have been proposed. Some of the most widely-used are the percentage of normal precipitation, the number of consecutive dry days (CDDs), the Palmer Drought Severity Index (PDSI), the Standardized Precipitation Evaporation Index (SPEI), the Standardized Precipitation Index (SPI), the Aridity Index (AI), the Standardized Runoff Index (SRI), the Supply–Demand Drought Index (SDDI), to name a few (WMO/GWP, 2016, and references therein). These indicators are usually based on station observations. Likewise, gridded observations, satellite-based products or reanalysis products are often used for assessing drought. In such cases, observational uncertainty due to the lack of reliable and consistent observations in the region should be taken into consideration (Zittis, 2018).
3.2.2 Observed trends and future projections
Observed droughts are mostly found to be driven by natural variability, yet the role of climate change in triggering or enhancing drought has increased in recent decades (Hoerling et al., 2012). A large number of studies based on observations or climate reconstructions investigated past trends of droughts in the eastern Mediterranean region. While the sign and significance of trends strongly depend on the period under consideration, the majority of analyses suggest an ongoing transition to future drier conditions. Based on wintertime precipitation reconstructions and observations, Lelieveld et al. (2012) provided evidence that the eastern Mediterranean dry period, which started in the early 1960s, was the driest period of the last 500 years. Recent high-impact droughts have thus received great attention; for example, the 15-year drought in the Levant (1998–2012) is the driest period in observational records, and it is very likely drier than any comparable period of the last 900 years (Cook et al., 2016). Nevertheless, considering the 20th century as a whole, it was one of the wettest periods over the late Holocene (Morin et al., 2019), highlighting the strong temporal variability and the dependence on the period under consideration when assessing past changes (Nicault et al., 2008). The recent severe Syrian drought has become more than twice as likely because of human interference in the climate system (Kelley et al., 2015). Philandras et al. (2011) identified decreasing precipitation trends in most of the Mediterranean regions during the period 1901–2009, particularly in 1951–2009. For the eastern part of the basin, they also highlighted a decrease in the number of rainy days. In the same context, Hoerling et al. (2012) analyzed several data sets and concluded that droughts in the region have shown an increasing trend, particularly over the last 20 years of the period 1902–2010. Nastos et al. (2013a) studied the spatiotemporal patterns of the Aridity Index in Greece for 1951–2000. They illustrated a progressive shift from the humid, towards the sub-humid and semi-arid class for eastern Greece. Similarly, Donat et al. (2014) analyzed station data in the Arabian region for 1951–2010. For the eastern Mediterranean, they highlight non-significant yet positive trends of dry spells using the CDD index over the period 1950–2017, in accordance with Yosef et al. (2019). Nonetheless, statistically significant negative trends for eastern Mediterranean wintertime precipitation and positive trends for CDD were reported by more recent studies (Seager et al., 2019; Zittis, 2018; Hochman et al., 2018c). Caloiero et al. (2018) identified significant negative trends for 12–24-month standardized precipitation index values over the eastern Mediterranean. Likewise, Güner Bacanli (2017) explored precipitation and drought trends in a number of stations in Turkey. The conclusions on sign and level of significance depend on the location and standardized precipitation index accumulation scale. Overall, the area covering the eastern Mediterranean and the Levant is considered a drought hotspot that has experienced a robust increase in drought frequency and severity, particularly over the last few decades, especially when considering the synergistic effect of temperature and evapotranspiration (cf. Figs. 4 and S1 in the Supplement; Spinoni et al., 2019).
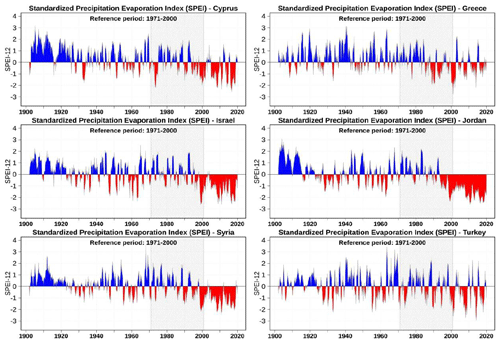
Figure 4Standardized Precipitation Evaporation Index (SPEI) anomalies for six selected countries in the eastern Mediterranean. The plot is based on the CRU TS4.04 gridded monthly precipitation and temperature data set (Harris et al., 2020). Positive values (blue) indicate wet periods, and negative values (red) indicate dry periods.
A number of studies have associated anthropogenic climate change with an expansion of the Hadley Cell in annual average and poleward shift of storm tracks that weaken the westerlies at mid-latitudes (e.g., Lu et al., 2007). Since Mediterranean cyclones are a major precipitation-producing weather system in the region (Pfahl et al., 2014; Flaounas et al., 2018b), this feedback mechanism induces regional drying (Seager et al., 2019). About 85 % of the area-averaged Mediterranean wet-season precipitation reduction is attributed to such atmospheric circulation responses (Zappa et al., 2015b). However, the significance of this response is still under debate (e.g., Shaw et al., 2016; Garfinkel et al., 2020). Precipitation projections for the eastern Mediterranean are mainly characterized by relatively low levels of significance and robustness (Lelieveld et al., 2016; Zittis et al., 2019). Mostly, when it comes to RCP8.5 (business-as-usual pathway) and middle-to-end-of-century estimations, a strong and significant precipitation decrease is projected (Samuels et al., 2018; Cherif et al., 2020; Hochman et al., 2019b). However, droughts are also driven by temperature (and thus evapotranspiration) increases, which are found to be quite robust. Future projections for drought risk, expressed in terms of the Palmer Drought Indices, indicate a significant decrease in soil moisture for all seasons, as well as increases in the severity and length of future droughts (Dubrovský et al., 2014; Liu et al., 2018). Based on global climate projections, Touma et al. (2015) underline that the spatial extent, occurrence and duration of exceptional droughts are projected to increase in subtropical regions (including the eastern Mediterranean) in the 21st century. Spinoni et al. (2020) utilized regional climate model output and created global SPEI projections. They concluded that the Mediterranean, including its eastern part, is among the global hotspot areas for severe droughts in the future. They also highlighted the role of temperature and evaporation in future events. Up to 60 % additional days of drought conditions, resulting in an increase of about 20 %–40 % in the number of dry years, are expected for the region (Prudhomme et al., 2014; Driouech et al., 2020). Climate projections based on a European modeling domain, which also includes parts of the eastern Mediterranean (Balkans and Anatolia), suggest a robust increase in the length (up to 3–4 additional weeks) and severity of extreme dry spells for the future (Jacob et al., 2014; Spinoni et al., 2018). Similarly, Tabari and Willems, (2018) expect less frequent rainy days and prolonged dry periods for the future in the eastern Mediterranean countries. High-resolution country-based projections (e.g., for Israel and Cyprus) also highlight that drought indicators, such as consecutive dry days, are projected to increase in future decades (Hochman et al., 2018c; Zittis et al., 2020). While most models agree on an increase in the frequency and severity of drought episodes over the eastern Mediterranean, there is still a large uncertainty depending on the chosen definition of drought, future socio-economic scenario and climate model (e.g., Cook et al., 2014; Dubrovský et al., 2014; Tramblay et al., 2020).
3.2.3 Societal impacts
Drought impacts are expected to increase in the future, in particular for developing countries in the southern and eastern parts of the Mediterranean (Tramblay et al., 2020). Socio-economic sectors and ecosystems affected by high-impact droughts include domestic water supply, agriculture, livestock production, leisure activities, hydroelectric power production, biodiversity and more. Therefore, the water–food–energy nexus in the broader Mediterranean region is disturbed in various ways when prolonged drought events occur (Lange, 2019; Markantonis et al., 2019). Moreover, parts of the eastern Mediterranean are characterized by pronounced inequalities, and the poor are expected to suffer most from climate change impacts on water and other resources (Waha et al., 2017). For example, the vulnerability of livestock production systems to droughts was recently demonstrated in northeastern Syria, where herders lost almost 85 % of their livestock because of the drought of 2005–2010 (Waha et al., 2017). The amount and quality of water resources are critically affected by prolonged droughts. Such recent events have led to irreversible salinization processes in the aquifers and negative ecological conditions in the Sea of Galilee, Israel (Inbar and Bruins, 2004). Furthermore, projected population and land-use changes are expected to exacerbate the effects of warmer and drier climatic conditions (Spinoni et al., 2020). If the internal water footprint (i.e., total volume of fresh water used to produce the goods and services consumed) of the eastern Mediterranean countries declines in line with precipitation. Moreover, the total water footprint of the region increases in line with population, by 2050 as much as half of the total water requirements will need to be provided through desalination and imported water (Chenoweth et al., 2011). The projected transition to warmer and drier bio-climatic conditions will severely affect agriculture and thus food production, which are particularly vulnerable to drought. Mediterranean crops, e.g., olives, vines and wheat, will be strongly influenced by the combined effect of summer droughts and heat stress (Sen et al., 2012; Constantinidou et al., 2016; Papadaskalopoulou et al., 2020). Interestingly, for some crops, agricultural production in high-altitude regions might be positively influenced. Still, for some eastern Mediterranean agroecosystems, the projected climatic pressure lies outside the limits of resilience (Daliakopoulos et al., 2017). Moreover, severe droughts can favor the preconditions for forest fires, predominantly during the warm and dry part of the year. Summer fires frequently rage across the Mediterranean, often intensified by high temperatures and droughts that are found to regulate fuel moisture (Turco et al., 2017a). Nevertheless, droughts also control fuel availability, making the relationships between fire activity and weather conditions more complex (Turco et al., 2017b). For eastern Mediterranean forests, days with critical fire risk, length of fire season, burned areas, etc. are expected to increase in the 21st century, mainly under business-as-usual pathways (Karali et al., 2014; Çolak and Sunar, 2020; Dupuy et al., 2020). Finally, limitations in water resources due to prolonged drought events and associated impacts are found to trigger or augment conflicts and disputes in the region (Gleick, 2014; Kelley et al., 2015). Regional economic, political, demographic and social drivers, as well as environmental stressors such as drought, could result in forced migration flows, and climate change acts as a trigger favorable to this direction (Black et al., 2011; Tabari and Willems, 2018; Abel et al., 2019). However, there is still a controversy as to how droughts may influence conflict and political unrest (e.g., Boas et al., 2019).
Surface winds arise from pressure gradients in the atmosphere and are a fundamental component of weather and climate. They are key in controlling air–sea and air–land exchanges of heat, water and chemical constituents and can pose an immediate societal threat due to direct damage of strong winds and wind gustiness (e.g., Klawa and Ulbrich, 2003; Pinto et al., 2012). Understanding the variability of winds is vital for estimating wind energy potential (Shata and Hanitsch, 2006; Hueging et al., 2013; Drobinski et al., 2018) and for managing air quality and health aspects (Georgiou et al., 2018), among many other factors. Understanding the mechanisms underlying the spatial–temporal variability of winds, their extremes and future trends is therefore key for understanding Earth systems interactions and for reducing societal risks from extreme events, while preparing for their future changes.
In the eastern Mediterranean, strong surface winds of velocities exceeding 20 m s−1 are typically associated with cyclones and prevail predominantly in the winter months. Such intensities can also be found in autumn and spring and are almost completely absent in the summer months according to 9 years of QuickSCAT data (Chronis et al., 2011) or by estimation of 10 m wind gust anomalies in the ERA-Interim reanalysis (Raveh-Rubin and Wernli, 2015). Using a process-based definition, namely 10 m wind speed exceedance of the 98th percentile in the vicinity of a cyclone, on average 3–4 extreme wind storm days occur per extended winter season in most eastern Mediterranean locations (Nissen et al., 2010, 2014).
Strong winds in the eastern Mediterranean are mostly westerly (or northwesterly/southwesterly) or easterly (Chronis et al., 2011). Naturally, as inferred from the marine or continental pathway of the air masses involved and the orientation of the easternmost Mediterranean coastline, strong westerly and easterly winds in the region differ substantially in their characteristics and impact (Fig. 5). Westerlies peak upon the passage of Mediterranean cyclones and are often accompanied by moist flow and heavy precipitation over land (see Sect. 3.1.1; Saaroni et al., 2010; Raveh-Rubin and Wernli, 2015; Martius et al., 2016; Berkovic et al., 2021). Strong easterlies, also peaking in winter, occur at ∼ 10 % yearly frequencies very locally in Israel, with wider regions experiencing such winds ∼ 1.4 % of the time (Saaroni et al., 1998). The easterly regime has a strong signature away from the Mediterranean coast, such as over the Judean and Samarian mountains, where the opposing sea breeze is uncommon (Fig. 5d; Saaroni et al., 1998; Berkovic, 2017).
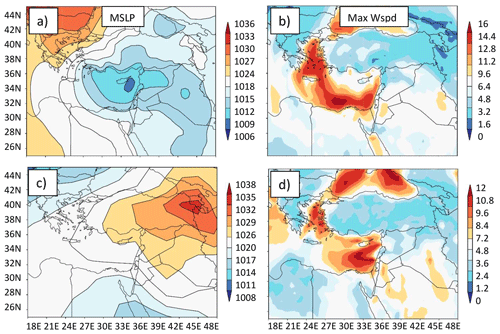
Figure 5The signature of the maximum daily 10 m wind speed (m s−1) for two eastern Mediterranean windstorms. Mean daily sea level pressure (MSLP, in hPa – left column) and maximum daily wind speed (Max Wspd, in m s−1 – right column) for a westerly wind storm on 8 January 2020 (a, b) and an easterly wind storm on 23 January 1986 (c, d). The figure is based on ERA5 reanalysis data (Hersbach et al., 2020).
An immediate impact of strong winds in coastal regions is the potential emergence of storm surges. In the eastern Mediterranean, typically 4–6 events per year occur, based on in situ data and numerical modeling of storm surges in Alexandria, Egypt (Cid et al., 2016). However, Androulidakis et al. (2015) showed that storm surges in Alexandria, manifested as sea level height anomalies, do not reach 20 cm and are thus more moderate compared to other coastal regions in the Mediterranean basin (e.g., Conte and Lionello, 2013). During these times, the wind direction is more often westerly and southerly compared to climatology when northerlies dominate. In Hadera, Israel, wind direction during high sea level periods is anomalously southerly and easterly compared to climatology, which is dominated by northerly and westerly winds (Androulidakis et al., 2015).
4.1 Physical understanding
Extreme surface winds emerge under variable large-scale atmospheric conditions from the synoptic to the mesoscale and can generally emerge or enhance through the interaction of the airflow with orography or the land–sea contrast (Ulbrich et al., 2012).
Upper quantiles of wind speeds at 10 m height based on 71 automated weather stations in Israel indicate that the highest wind speeds occur preferentially under cyclonic synoptic regimes (Osetinsky-Tzidaki and Venger, 2020). Nissen et al. (2010) compiled a climatology of windstorms induced by cyclones for the Mediterranean and found Cyprus to be the secondary region of wind-inducing cyclones in the Mediterranean, after the Gulf of Genoa. On average, 3–4 wind extreme days (see definition above) occur per extended winter season, with the peak in wind located between Cyprus and Alexandria, ∼ 400–800 km south of the mean Cyprus Low center (Fig. 5a, b; Nissen et al., 2010).
An objective classification of the winter surface winds in Israel was shown to be strongly linked to the regional circulation induced by the dominant synoptic systems (Berkovic, 2017). In a systematic classification of radiosonde data from Bet-Dagan, Israel, Berkovic et al. (2021) distinguished recurring winter regimes of boundary-layer profiles including wind magnitude and direction. The strongest surface winds were southwesterly, with mean magnitudes of 10 m s−1, followed by less extreme (north) westerly (3–4 m s−1) and more moderate easterly or northeasterly directions. Generally, winds under a southwesterly regime were directly linked to the location of the Cyprus Low and the pressure gradient it induces, situations combined with cold temperatures and heavy precipitation (Fig. 5a, b; see Sects. 2.1 and 3.1.1).
Based on the 10 most extreme large-scale wind gust events in the eastern Mediterranean, Raveh-Rubin and Wernli (2015) found winds peaking upon a substantial regional drying (up to 4 kg m−2 reduction of total column water). Cooling of the lower troposphere by 6 K and strengthening of a northerly wind component (i.e., generally westerly winds become northwesterly) over the course of the 36 h led to peak winds. Such a change is a result of the passage of an upper-tropospheric trough and a surface cyclone ahead of a ridge in the upper troposphere. Focusing on a combined extreme wind and precipitation case in 11 December 2010, Raveh-Rubin and Wernli (2016) showed that, the air masses of strongest winds exhibit a backward-trajectory pathway reminiscent of the cold conveyor belt concept, i.e., a lower-tropospheric jet turning cyclonically around the cyclone center on its cold side (e.g., Smart and Browning, 2014). In addition, wind gust hotspots may also prevail due to deep moist convection embedded in the cyclone's cold air mass that destabilizes above the warm Mediterranean Sea (Ziv et al., 2009; Raveh-Rubin and Wernli, 2016).
In contrast, strong easterlies occur under dry and relatively warm winter conditions induced by a high-pressure system to the northeast, often accompanied by the surface Red Sea Trough (Fig. 5c, d; Saaroni et al., 1998; Berkovic, 2017). During strong easterlies, quasi-stationary anticyclones with variable locations over Asia (or at times over Eastern Europe) exhibit ridge disturbances into the Levant (Saaroni et al., 1996).
Interestingly, high sea level in Alexandria and Hadera is only weakly directly correlated with wind speed or sea level pressure (Androulidakis et al., 2015). Although the wind influence on surges in the eastern Mediterranean is weaker compared to northwestern Mediterranean areas, surges in Alexandria, Egypt, and İskenderun, Turkey, are associated with increased frequency of Mediterranean cyclones (Lionello et al., 2019). In their study, based on hindcasts of the 100 most extreme surge events in Alexandria and İskenderun using a barotropic ocean circulation model forced by ERA-Interim downscaled fields, cyclones were frequent in the southeastern and northeastern parts of the Mediterranean, respectively. Detailed mechanistic association between anomalous surface winds, storm surges and the relation to the life cycles of weather systems in the region is generally underexplored.
4.2 Observed trends and future projections
A decrease in surface wind speeds over land areas in the Northern Hemisphere has been observed in recent decades, termed wind stilling (McVicar et al., 2012). In this respect, the eastern Mediterranean is no exception, displaying reductions between 0.001 m s−1 yr−1 in Greece (1959–2001; Papaioannou et al., 2011) and 0.04 m s−1 yr−1 in Cyprus (1982–2002; Jacovides et al., 2002). However, no consensus has been reached regarding the extreme winds (Drobinski et al., 2018). Indeed, it should be noted that observed trends of extreme winds have generally received less attention in the literature with respect to, e.g., extreme temperatures and precipitation.
A decrease of 2–3 m s−1 by the end of the 21st century was indicated for the 99.5 percentiles of the 925 hPa winds using the ECHAM5 global climate model (Bengtsson et al., 2009). The trend is consistent with earlier projections of a decrease in intense windstorms in the region (e.g., Pinto et al., 2007). As already discussed, a significant reduction in winter cyclone activity is expected in the Mediterranean region and in the eastern Mediterranean in particular (e.g., Zappa et al., 2015a; Hochman et al., 2018b). However, uncertainty remains with regard to future projections of the most intense cyclones, with some studies indicating a decrease in cyclone intensities (e.g., Pinto et al., 2007) or frequency (e.g., Nissen et al., 2014; Hochman et al., 2020d). Nissen et al. (2014) focus on windstorms associated with cyclones, suggesting a decrease by 0.5–1 extreme windstorm days per winter season by the end of the 21st century from the current mean of ∼ 4 d. The decrease is attributed to reduced cyclone intensities and emerges despite a local increase in cyclone numbers in the eastern Mediterranean in their models (Nissen et al., 2014). However, the return periods of the most severe storms do not change, suggesting that, despite their general decrease, windstorms remain an important risk in the region (Nissen et al., 2014). Thus, regional projections of strong winds may be highly threshold dependent, warranting continued investigation.
It should be noted that the seasonal distribution of winds may change under future climate conditions, potentially redefining what a typical season is in terms of mean and extreme winds. Hochman et al. (2018b) demonstrated a 49 % lengthening of the summer season in terms of the occurrence of the Persian Trough (Fig. 2e, f; see Sect. 2.1) by the end of the 21st century, consistent with a projected increase in the occurrence and intensity of the Etesian winds (Hueging et al., 2013; Tobin et al., 2015; Mömken et al., 2018; Dafka et al., 2019).
Multiple numerical experiments under future scenarios suggest that mean and peak sea level height trends in the eastern Mediterranean (especially in the northeastern corner) are strongly controlled by sea level pressure changes, while mean sea level height is additionally affected by changes of winds (Androulidakis et al., 2015). Therefore, the expected reduction of storms during the 21st century suggests a reduction in the area susceptible to sea level rise extremes (Androulidakis et al., 2015), further suggesting decreasing storm surge rates of ∼ 2 % (Cid et al., 2016).
4.3 Societal impacts
Strong moist winds in winter, often occurring in combination with heavy precipitation, pose the highest societal risk from severe weather in the region (e.g., Llasat et al., 2010), with human casualties and widespread damage. Easterly winds impact agriculture significantly due to their unusual dryness, with warm advection increasing evaporation rates and cold advection often leading to frost (Saaroni et al., 1996). Occasionally, easterlies are conducive to air pollution, intensifying forest fires, and dust and sand storms (Saaroni et al., 1998). There is a lack of systematic studies estimating losses due to winds, and wind power potential, especially considering future trends over the southeastern part of the Mediterranean (Drobinski et al., 2018). Indeed, the northwestern part of the region has received a relatively large amount of attention (e.g., Hueging et al., 2013; Tobin et al., 2015; Mömken et al., 2018). Altogether, the different hazards imposed by surface winds and their tight involvement in planning decisions necessitates a better understanding of the underlying mechanisms controlling their variability and trends to better predict extreme wind events on weather and climate scales.
5.1 Physical understanding
The term “compound extremes” refers to the combination of multiple extreme events or of multiple drivers that lead to an extreme. Crucially, their combined impact often exceeds the linear sum of the impacts of their components (Zscheischler and Seneviratne, 2017). Some authors extend the term “compound” to include temporally clustered or simultaneous but geographically remote events (e.g., Vahedifard, 2016; Baldwin et al., 2019). Here, we focus on the conventional case of spatially and temporally co-occurring drivers or extremes, such as heavy precipitation and strong storm surges leading to extreme flooding or co-occurring high temperatures and high atmospheric humidity. In the latter example, the heat stress, and thus the impacts on the local population, will be more severe than if a very hot and a very humid day had occurred independent of each other (e.g., Sherwood and Huber, 2010).
In the eastern Mediterranean, the most impactful compound extremes are those resulting from a combination of temperature and/or hydrological extremes, although other extremes may also show compounding behavior, such as windstorms combined with heavy precipitation (see Sect. 4.1; Nissen et al., 2010; Raveh-Rubin and Wernli, 2015; Catto and Dowdy, 2021). The eastern Mediterranean routinely experiences very high summertime temperatures (see Sect. 2), and although the summer climate is generally dry, high ambient humidity levels can be reached locally (e.g., Unal et al., 2013). From a mesoscale atmospheric perspective, hot and humid summertime extremes are favored by a stable low-level atmosphere, which traps near-surface moisture (Ziv and Saaroni, 2011). Regional sea surface temperatures may also play an important role by modulating air temperatures through the strengthening or maintenance of anticyclonic circulations associated with descending motions and cloud-free skies (see Sect. 2; Unal et al., 2013) and by controlling moisture supply. On a larger scale, the hot and humid extremes are associated with the advection of air masses from southern continental Europe, which undergo adiabatic descent over the eastern Mediterranean, and the presence of an upper-level trough (Hochman et al., 2021a). However, we note that the latter authors based their analysis on an index combining temperature and humidity with additional atmospheric parameters, such as the height of the marine inversion.
Although comparatively rare, wintertime cold spells also have severe impacts on the eastern Mediterranean (see Sect. 2) and can be associated with heavy precipitation in the form of snowfall. Such events are often favored by a concurrent upper-level anticyclone over northern or western Europe and a cyclone over the eastern Mediterranean, which together drive the transport of cold air masses to the region, whose moisture and stability properties are affected by the land–sea distribution they encounter along their path (e.g., Alpert and Reisin, 1986; Tayanç et al., 1998). However, the details of the cold air mass transport can differ significantly between individual cold and snowy episodes (Fig. 1b). The early winter episode of 1982 described by Alpert and Reisin (1986), which led to one of the few recorded November snowfall events in Israel, was associated with northerly advection. In December 2013, Winter Storm Alexa, associated with heavy snowfall in Jerusalem, displayed a similar advection pathway linked to a Cyprus Low type circulation (Fig. 2a, b; Hochman et al., 2020d). In contrast, the notable March 1987 cold spell and heavy snowfall, which was particularly severe in Greece and Turkey (as well as in much of the Balkan Peninsula), was associated with a northeasterly advection (Tayanç et al., 1998). This characteristic is shared by the majority of snowfall events in Athens over the second half of the 20th century (Houssos et al., 2007). A climatology of cold spells leading to snowfall in Jerusalem shows yet another pattern, with a median northwesterly advection pathway (Hochman et al., 2020d).
Shifting the focus to hydrological extremes, the eastern Mediterranean region is vulnerable to both compound drought and compound flooding episodes. One of the chief sources of compound flooding is the combination of heavy precipitation and strong storm surges in coastal areas. The Mediterranean in general and the eastern Mediterranean in particular emerge as regions with a high probability of compound flooding occurrence, associated with the presence of deep low-pressure systems in the region (Bevacqua et al., 2019). Compound drought events are mainly investigated through multivariate drought indices (see Sect. 3.2.1). An intuitive example of a compound drought would be the case where a precipitation deficit co-occurs with unusually high temperatures (e.g., Vogel et al., 2021).
5.2 Observed trends and future projections
The frequency and duration of heat waves has been increasing in the eastern Mediterranean in recent decades, a trend which is projected to continue in the future against the background of global warming (Fig. 3a; see Sect. 2.2). Relative humidity is also expected to show an increasing trend in the region due to increased lower-level stability (Ziv and Saaroni, 2011). Assuming no change in the statistical relation between temperature and humidity, these two concomitant increases point to an increased occurrence and/or severity of hot and humid extremes in the eastern Mediterranean. These changes are already visible in the observational record (Ziv and Saaroni, 2011; Unal et al., 2013), and there is evidence for a generalized future increase in heat stress risk across the eastern Mediterranean (e.g., Ahmadalipour and Moradkhani, 2018), with coastal areas being particularly exposed (Diffenbaugh et al., 2007). On the other hand, the frequency of cold spells is projected to decrease globally (albeit less than may be expected), and in this respect the eastern Mediterranean will be no exception (see Sect. 2.2). We are, however, not aware of any studies focusing specifically on future projections of compound cold an snowy events over the region.
Large uncertainties still impair our understanding of changes in hydrological extremes over the eastern Mediterranean (see Sect. 3). Models disagree on future changes in compound flooding over the region and range from moderate increases to strong decreases in the return period of such events (Bevacqua et al., 2019). Observed trends in multivariate drought indices have been the subject of heated discussion in the literature (e.g., Seneviratne, 2012), and studies focusing on the eastern Mediterranean reflect the large uncertainty on the topic (see Sect. 3.2.2). One projection that appears more robust is that of an increased co-occurrence of compound precipitation deficits and high temperatures, which is primarily driven by the increasing frequency and severity of heat waves (Vogel et al., 2021). Indeed, a positive trend in such events has been observed across most of the eastern Mediterranean in recent decades (Mukherjee and Mishra, 2021).
5.3 Societal impacts
Compound extremes in the eastern Mediterranean are already imposing a heavy socio-economic toll. Combined temperature–humidity extremes in Greece reached warning levels for public health and the ability of the workforce to carry out normal tasks already in the 1980s (Giles et al., 1990). Although in warm regions one may expect the local population to have developed some degree of acclimatization, cities like Tel-Aviv – and to a lesser degree Athens – display an average summertime temperature–humidity level that is very close to the threshold beyond which an impact on mortality can be observed. This makes these cities vulnerable to extreme temperature–humidity events already under current climate conditions (Leone et al., 2013). Studies further point to a manifold increase in mortality risk due to temperature–humidity extremes across the eastern Mediterranean by the end of the century, even under moderate climate change scenarios (Ahmadalipour and Moradkhani, 2018). On the opposite end of the scale, cold and snowy events can also have severe impacts on the eastern Mediterranean. For example, Winter Storm Alexa in 2013 (Israel Meteorological Service, 2013) ranked as the costliest natural disaster in the region, with an estimated cost of USD 100 million. Compound floods and compound droughts (see Sect. 5.1) in the eastern Mediterranean have repeatedly had detrimental effects on the local population. The former have the potential to damage coastal infrastructure and environments (e.g., the 2010 compound flood in Alexandria; see also Ismail et al., 2012). The latter can compromise food security and may have a role in precipitating regional conflicts (see Sect. 3.2.3). As most studies focusing on societal impacts of droughts do not explicitly consider the compound nature of drought, we discuss these in depth in Sect. 3.2.2.
As evident from the above discussion, there is a considerable literature on compound extremes in the eastern Mediterranean. However, a large number of studies only implicitly consider the compound nature of the extremes, for example using multivariate heat stress or drought indices, and do not focus on the dependence structure of the different variables or drivers of the extremes in current and future climates. A clearer focus on the role of concurrent drivers or anomalies in several variables may help in advancing our understanding of compound extremes in the region.
Extreme weather in the eastern Mediterranean has detrimental effects on society and ecosystems. Here, we provide a review of the state-of-the-art and current research knowledge gaps on this subject. We specifically focus on the physical processes that drive extreme weather, the observed trends and future projections of such events and finally the societal impacts these events may have.
Extreme weather in the eastern Mediterranean is connected with regional synoptic systems and their interplay with the large-scale atmospheric flow. The region has experienced repeated extreme heat waves in the recent past (Kuglitsch et al., 2010), and their frequency, duration and intensity are projected to increase in the coming decades (Fig. 3a; e.g., Seneviratne 2012; Lelieveld et al., 2016; Hochman et al., 2018a). On the other hand, the frequency and duration of winter cold spells are projected to decrease in the coming decades (Fig. 3b; Sillmann et al., 2013). Still, it should be noted that eastern Mediterranean cold spells have received comparatively little consideration in the literature, perhaps due to the naive assumption that they will no longer occur in a warmer climate.
The majority of extreme weather events, including heavy precipitation, cold spells, floods and windstorms, can be associated with Cyprus Lows or active Red Sea Troughs (Figs. 1b, c and 2a, b; Alpert and Reisin, 1986; Nissen et al., 2010). Similar to other Mediterranean cyclones, Cyprus Lows have been projected to significantly decrease in frequency, persistence and accompanying daily precipitation amounts under increased greenhouse gas concentration pathways (e.g., Hochman et al., 2018a, 2020b). Active Red Sea Troughs have recently received ample attention as there is an ongoing debate about how an increase in greenhouse gas concentrations will influence their frequency and intensity (Alpert et al., 2004; Peleg et al., 2015a; Saaroni et al., 2020; Hochman et al., 2021b; Marra et al., 2021a). We note here that there is relatively little literature related to the physical understanding, observed trends and future projections of wind extremes in the southeastern part of the eastern Mediterranean.
Compound extremes in the eastern Mediterranean are often linked either with very hot and humid conditions during summer, especially close to the Mediterranean coast, or cold and wet episodes during winter. Hydrological compound extremes include compound droughts and compound coastal flooding. Hot and humid extremes are projected to increase in frequency and intensity (see Sect. 5.2). There is considerable uncertainty in trends in compound droughts and floods, although some studies point to increasing trends. To the best of our knowledge, studies focusing specifically on future projections of compound cold and wet events over the eastern Mediterranean are not yet available.
Extreme weather events impose heavy socio-economic tolls (IPCC, 2021). These are further aggravated in vulnerable regions, such as the eastern Mediterranean (e.g., Lelieveld et al., 2012). We identify the following four key means to increase societal resilience to extreme weather: (i) reduce the probability of extreme weather by timely mitigation of hazardous climate change; (ii) improve the ability to forecast extreme weather and its impacts using conventional and/or novel techniques and early warning systems (e.g., Hochman et al., 2020d, 2021a; Merz et al., 2020); (iii) expand the database related to the impacts of extreme weather to support a better mapping of vulnerabilities (e.g., Merz et al., 2020); and (iv) develop adaptation strategies to cope with the adverse impacts extreme events may have on society, infrastructure, and human-controlled ecosystems. In respect to the latter two points, some specific knowledge gaps have been identified. These mainly relate to the impacts extreme weather may have on mortality, morbidity and infrastructure in the eastern Mediterranean. We believe that societal resilience to extreme weather in the region can properly be achieved only by true interdisciplinary cross-border collaborations in spite of recurring political turmoil (e.g., Hochman et al., 2020a, c; Negev et al., 2021).
We provide an overview of the research conducted and main knowledge gaps relating to extreme weather and its societal impacts in the eastern Mediterranean. The following four key open questions, immediately relevant for the eastern Mediterranean countries, have been identified. They should be considered as suggestions for future research initiatives and are therefore framed as overarching questions.
-
Can we skillfully predict the onset, duration and intensity of extreme weather events across time scales?
The predictability of extreme weather events across scales from daily to sub-seasonal to decadal and beyond is of special interest. Depending on the timescale, the focus should be on single case studies and process understanding or on analyzing the long-term statistical properties of extreme weather events.
-
How will extreme weather affect eastern Mediterranean countries?
The direct and indirect influence that extreme weather may have on eastern Mediterranean countries is of particular interest. This question calls for an interdisciplinary collaboration between meteorologists, climatologists, hydrologists and impact scientists to study the links between extreme weather and its influence on different sectors in the eastern Mediterranean.
-
Can we predict the impacts extreme weather inflicts on society and infrastructure across time scales?
The impacts extreme weather events may inflict depend on not only the events themselves but also the local to regional economic resilience and adaptation measures put in place. These may differ strongly from country to country in the eastern Mediterranean. Therefore, providing regional impact forecasts across timescales is of particular importance. Such forecasts may provide strategic guidelines for national and regional adaptation plans.
-
How should policy measures change to cope with the impact extreme weather may inflict upon society?
Since the full impact of climate change on eastern Mediterranean countries is yet to come, recommendations in the public sector are not tailored to future changes in extreme weather occurrence and/or intensity. Data-driven recommendations for specific interventions are therefore of the utmost importance.
While we have pointed to some key open questions in this review, we acknowledge that enormous advances in the understanding of extreme weather over the eastern Mediterranean have already been made by the studies we refer to and by many others we could not include. We hope that this review can be considered as a framework for future research on the topic.
The analysis in this paper is based on the European Center for Medium-range Weather Forecasting (ECMWF) ERA5 reanalysis (https://www.ecmwf.int/en/forecasts/datasets/reanalysis-datasets/era5; Hersbach et al., 2020), the Coupled Model Intercomparison Project phase 5 models (Table S1; https://esgf-node.llnl.gov/projects/cmip5/; Taylor et al., 2012), and the Climatic Research Unit (CRU) Time Series (TS) version 4.04 of high-resolution gridded data of month-by-month variation in climate (https://crudata.uea.ac.uk/cru/data/hrg/cru_ts_4.04/; Harris et al., 2020).
The supplement related to this article is available online at: https://doi.org/10.5194/esd-13-749-2022-supplement.
The original idea for the paper was developed by AH, GM and JGP. The concept was developed together with all co-authors. AH wrote the abstract, introduction (Sect. 1), summary and knowledge gaps (Sect. 6), and key open questions (Sect. 7). YY wrote the part addressing temperature extremes (Sect. 2). FM wrote the section on heavy precipitation (Sect. 3.1), and GZ wrote the part on droughts (Sect. 3.2). SRR wrote the passages on wind extremes (Sect. 4). GM wrote the section on compound extremes (Sect. 5). AH prepared Figs. 2, 3 and 5. GZ prepared Figs. 4 and S1. GM prepared Fig. 1 with contributions from all co-authors. All authors contributed with discussions and revisions.
At least one of the (co-)authors is a member of the editorial board of Earth System Dynamics. The peer-review process was guided by an independent editor, and the authors also have no other competing interests to declare.
Publisher's note: Copernicus Publications remains neutral with regard to jurisdictional claims in published maps and institutional affiliations.
The authors acknowledge the European Center for Medium Range Weather Forecasting for making the ERA5 reanalysis freely available. We further acknowledge the Climatic Research Unit of the University of East Anglia for making high-resolution observations freely available. This study is part of the cooperation in the international virtual institute DESERVE (Dead Sea Research Venue). This work is a contribution to the hydrological cycle in the Mediterranean Experiment (HyMex) and COST MEDCYCLONES: European Network for Mediterranean Cyclones in weather and climate. Assaf Hochman is grateful for technical support provided by the Hebrew University of Jerusalem.
This research has been supported by the German Helmholtz Association (“Changing Earth”
program) in the case of Assaf Hochman. Joaquim G. Pinto was supported by the AXA Research Fund. Gabriele Messori received funding from the European Research Council (ERC) under the European Union's
Horizon 2020 research and innovation program (grant agreement no. 948309,
CENÆ Project). Francesco Marra was partly supported by the National Research Council
of Italy and by the Institute of Atmospheric Sciences and Climate
(CNR-ISAC). Georgios Zittis was supported by the EMME-CARE project, which has received
funding from the European Union's Horizon 2020 Research and Innovation
Programme (grant no. 856612) and matching co-funding from the
Government of the Republic of Cyprus.
The article processing charges for this open-access publication were covered by the Karlsruhe Institute of Technology (KIT).
This paper was edited by Stefan Dekker and reviewed by two anonymous referees.
Abdallah, C.: Assessment of erosion, mass movements, and flood risk in Lebanon, in: Review and Perspectives of Environmental Studies in Lebanon, National Council for Scientific Research (CNRS)-Beirut, Lebanon, edited by: Kouyoumjian, H. and Ham, M., 2012.
Abel, G. J., Brottrager, M., Crespo Cuaresma, J., and Muttarak, R.: Climate, conflict and forced migration, Global Environ. Chang., 54, 239–249, https://doi.org/10.1016/j.gloenvcha.2018.12.003, 2019.
Ahlborn, M., Armon, M., Ben Dor, Y., Neugebauer, I., Schwab, M. J., Tjallingii, R., Shoqeir, J. H., Morin, E., Enzel., Y., and Brauer, A.: Increased frequency of torrential rainstorm during a regional late Holocene eastern Mediterranean drought, Quaternary Res., 89, 425–431, https://doi.org/10.1017/qua.2018.9, 2018.
Ahmadalipour, A. and Moradkhani, H.: Escalating heat-stress mortality risk due to global warming in the Middle East and North Africa (MENA), Environ. Int., 117, 215–225, https://doi.org/10.1016/j.envint.2018.05.014, 2018.
Ajjur, S. B. and Riffi, M. I.: Analysis of the observed trends in daily extreme precipitation indices in Gaza Strip during 1974–2016, Int. J. Climatol., 40, 6189–6200, https://doi.org/10.1002/joc.6576, 2020.
Almazroui, M., Awad, A. M., Islam, N. M., and Al-Khalaf, A. K.: A climatological study: wet season cyclone tracks in the East Mediterranean region, Theor. Appl. Climatol., 120, 351–365, https://doi.org/10.1007/s00704-014-1178-z, 2015.
Alpert, P. and Reisin, T.: An early winter polar air mass penetration to the eastern Mediterranean, Mon. Weather Rev., 114, 1411–1418, https://doi.org/10.1175/1520-0493(1986)114<1411:aewpam>2.0.co;2, 1986.
Alpert, P. and Shay-El, Y.: The moisture source for the winter cyclones in the eastern Mediterranean, Isr. Meteor. Res. Pap., 5, 20–27, 1994.
Alpert, P. and Ziv, B.: The Sharav Cyclone: Observations and some theoretical considerations, J. Geophys. Res., 94, 18495–18514, https://doi.org/10.1029/JD094iD15p18495, 1989.
Alpert, P., Price, C., Krichak, S. O., Ziv, B., Saaroni, H., Osetinsky, I., Barkan, J., and Kishcha, P.: Tropical tele-connections to the Mediterranean climate and weather, Adv. Geosci., 2, 157–160, https://doi.org/10.5194/adgeo-2-157-2005, 2005.
Alpert, P., Ben-Gai, T., Baharad, A., Benjamini, Y., Yekutieli, D., Colacino, M., Diodato, L., Ramis, C., Homar, V., Romero, R., Michaelides, S., and Manes, A.: The paradoxical increase of Mediterranean extreme daily rainfall in spite of decrease in total volumes, Geophys. Res. Lett., 29, 1536, https://doi.org/10.1029/2001GL013554, 2002.
Alpert, P., Osetinsky, I., Ziv, B., and Shafir, H.: Semi-objective classification for daily synoptic systems: Application to the eastern Mediterranean climate change, Int. J. Climatol., 24, 1001–1011, https://doi.org/10.1002/joc.1036, 2004.
Alpert, P., Krichak, S. O., Shafir, H., Haim, D., and Osetinsky, I.: Climatic trends to extremes employing regional modeling and statistical interpretation over the E. Mediterranean, Global Planet. Change., 63, 163–170, https://doi.org/10.1016/j.gloplacha.2008.03.003, 2008.
Amponsah, W., Ayral, P.-A., Boudevillain, B., Bouvier, C., Braud, I., Brunet, P., Delrieu, G., Didon-Lescot, J.-F., Gaume, E., Lebouc, L., Marchi, L., Marra, F., Morin, E., Nord, G., Payrastre, O., Zoccatelli, D., and Borga, M.: Integrated high-resolution dataset of high-intensity European and Mediterranean flash floods, Earth Syst. Sci. Data, 10, 1783–1794, https://doi.org/10.5194/essd-10-1783-2018, 2018.
Amponsah, W., Marra, F., Marchi, L., Roux, H., Braud, I., and Borga, M.: Objective analysis of envelope curves for peak floods of European and Mediterranean flash floods, in: Climate Change, Natural Hazards and Adaptation Option: Handling the impacts of a changing climate, edited by: Leal Filho, W., Nagy, G., Borga, M., and Chávez Muñoz, P. D., Magnuszewski A, Springer, Cham, https://doi.org/10.1007/978-3-030-37425-9_14, 2020.
Androulidakis, Y. S., Kombiadou, K. D., Makris, C. V., Baltikas, V. N., and Krestenitis, Y. N.: Storm surges in the Mediterranean Sea: Variability and trends under future climatic conditions, Dynam. Atmos. Oceans, 71, 56–82, https://doi.org/10.1016/j.dynatmoce.2015.06.001, 2015.
Armon, M., Dente, E., Smith, J. A., Enzel, Y., and Morin, E.: Synoptic-scale control over modern rainfall and flood patterns in the Levant drylands with implications for past climates, J. Hydrometeorol., 19, 1077–1096, https://doi.org/10.1175/JHM-D-18-0013.1, 2018.
Armon, M., Morin, E., and Enzel, Y.: Overview of modern atmospheric patterns controlling rainfall and floods into the Dead Sea: Implications for the lake's sedimentology and palaeohydrology, Quaternary Sci. Rev., 216, 58–63, https://doi.org/10.1016/j.quascirev.2019.06.005, 2019.
Armon, M., Marra, F., Enzel, Y., Rostkier-Edelstein, D., and Morin, E.: Radar-based characterisation of heavy precipitation in the eastern Mediterranean and its representation in a convection-permitting model, Hydrol. Earth Syst. Sci., 24, 1227–1249, https://doi.org/10.5194/hess-24-1227-2020, 2020.
Armon, M., Marra, F., Enzel, Y., Rostkier-Edelstein, D., Garfinkel, C. I., Adam, O., Dayan, U., and Morin, E.: Reduced rainfall in future heavy precipitation events related to contracted rain area despite increased rain rate, Earth's Future, 10, e2021EF002397, https://doi.org/10.1029/2021EF002397, 2022.
Avni, Y., Lensky, N., Dente, E., Shviro, M., Arav, R., Gavrieli, I., Yechieli, Y., Abelson, M., Lutzky, H., Filin, H., Haviv, I., and Baer, G.: Self-accelerated development of salt karst during flash floods along the Dead Sea Coast, Israel, J. Geophys. Res.-Earth, 121, 17–38, https://doi.org/10.1002/2015JF003738, 2016.
Awad, A. M. and Almazroui, M.: Climatology of the winter Red Sea trough, Atmos. Res., 182, 20–29, https://doi.org/10.1016/j.atmosres.2016.07.019, 2016.
Baldi, M., Dalu, G., Maracchi, G., Pasqui, M., and Cesarone, F.: Heat waves in the Mediterranean: a local feature or a larger-scale effect?, Int. J. Climatol., 26, 1477–1487, https://doi.org/10.1002/joc.1389, 2006.
Baldwin, J. W., Dessy, J. B., Vecchi, G. A., and Oppenheimer, M.: Temporally compound heat wave events and global warming: An emerging hazard, Earth's Future, 7, 411–427, https://doi.org/10.1029/2018ef000989, 2019.
Ballester, J., Achebak, H., Herrmann, F., Robine, J., and Rodó, X.: Trends in heat-attributable vulnerability and mortality in Europe: role of macroeconomic growth during the Great Recession, Environmental Epidemiology, 3, 21, https://doi.org/10.1097/01.ee9.0000605844.32533.87, 2019.
Barcikowska, M. J., Kapnick, S. B., Krishnamurty, L., Russo, S., Cherchi, A., and Folland, C. K.: Changes in the future summer Mediterranean climate: contribution of teleconnections and local factors, Earth Syst. Dynam., 11, 161–181, https://doi.org/10.5194/esd-11-161-2020, 2020.
Barredo, J. I.: Major flood disasters in Europe: 1950–2005, Nat. Hazards, 42, 125–148, https://doi.org/10.1007/s11069-006-9065-2, 2007.
Baseer, M. N., Awad, A. M., and Almazroui, M.: Climatology of the spring Red Sea trough. Int. J. Climatol., 2019, 1–16, https://doi.org/10.1002/joc.6069, 2019.
Basu, R. and Samet, J. M.: Relation between elevated ambient temperature and mortality: A review of the epidemiologic evidence, Epidemiol. Rev., 24, 190–202, 2002.
Belachsen, I., Marra, F., Peleg, N., and Morin, E.: Convective rainfall in a dry climate: relations with synoptic systems and flash-flood generation in the Dead Sea region, Hydrol. Earth Syst. Sci., 21, 5165–5180, https://doi.org/10.5194/hess-21-5165-2017, 2017.
Ben Dor, Y., Armon, M., Ahlborn, M., Morin, E., Erel, Y., Brauer, A., Schwab, M. J., Tjallingii, R., and Enzel, Y: Changing flood frequencies under opposing late Pleistocene eastern Mediterranean climates, Sci. Rep.-UK, 8, 8445, https://doi.org/10.1038/s41598-018-25969-6, 2018.
Bengtsson, L., Hodges, K. I., and Keenlyside, N.: Will extratropical storms intensify in a warmer climate?, J. Climate, 22, 2276–2301, https://doi.org/10.1175/2008jcli2678.1, 2009.
Benito, G., Macklin, M. G., Zielhofer, C., Jones, A. F., and Machado, M. J.: Holocene flooding and climate change in the Mediterranean, Catena, 130, 13–33, https://doi.org/10.1016/j.catena.2014.11.014, 2015.
Ben-Zvi, A.: Rainfall intensity–duration–frequency relationships derived from large partial duration series, J. Hydrol., 367, 104–114, https://doi.org/10.1016/j.jhydrol.2009.01.007, 2009.
Berkovic, S.: Winter wind regimes over Israel using self-organizing maps, J. Appl. Meteorol. Clim., 56, 2671–2691, https://doi.org/10.1175/jamc-d-16-0381.1, 2017.
Berkovic, S., Mendelsohn, O. Y., Ilotoviz, E., and Raveh-Rubin, S.: Refinement of Eastern Mediterranean winter climatological regimes from self-organizing map analysis of boundary layer profiles, Int. J. Climatol., 4, 3317–3338, https://doi.org/10.1002/joc.7021, 2021.
Bevacqua, E., Maraun, D., Vousdoukas, M. I., Voukouvalas, E., Vrac, M., Mentaschi, L., and Widmann, M.: Higher probability of compound flooding from precipitation and storm surge in Europe under anthropogenic climate change, Science Advances, 5, eaaw5531, https://doi.org/10.1126/sciadv.aaw5531, 2019.
Black, E., Blackburn, M., Harrison, G., Hoskins, B., and Methven, J.: Factors contributing to the summer 2003 European heatwave, Weather, 59, 217–223, https://doi.org/10.1256/wea.74.04, 2004.
Black, R., Adger, W. N., Arnell, N. W., Dercon, S., Geddes, A., and Thomas, D.: The effect of environmental change on human migration, Global Environ. Chang., 21, S3–S11, https://doi.org/10.1016/j.gloenvcha.2011.10.001, 2011.
Boas, I., Farbotko, C., Adams, H., Sterly, H., Bush, S., van der Geest, K., Wiegel, H., Ashraf, H., Baldwin, A., Bettini, G., Blondin, S., de Bruijn, M., Durand-Delacre, D., Fröhlich, C., Gioli, G., Guaita, L., Hut, E., Jarawura, F. X., Lamers, M., Lietaer, S., Nash, S. L., Piguet, E., Rothe, D., Sakdapolrak, P., Smith, L., Tripathy Furlong, B., Turhan, E., Warner, J., Zickgraf, C., Black, R., and Hulme, M.: Climate migration myths, Nat. Clim. Change, 9, 901–903, 2019.
Borga, M., Stoffel, M., Marchi, L., Marra, F., and Jacob, M.: Hydrogeomorphic response to extreme rainfall in headwater systems: flash floods and debris flows, J. Hydrol., 518, 194–205, https://doi.org/10.1016/j.jhydrol.2014.05.022, 2014.
Borga, M., Comiti, F., Ruin, I., and Marra, F.: Forensic analysis of flash flood response, WIRES Water, 6, e1338, https://doi.org/10.1002/wat2.1338, 2019.
Boucek, R. E., Gaiser, E. E., Liu, H., and Rehage, J. S.: A review of subtropical community resistance and resilience to extreme cold spells, Ecosphere, 7, e01455, https://doi.org/10.1002/ecs2.1455, 2016.
Brikas, D. P., Karacostas, T. S., Pennas, P. J., and Flocas, A. A.: The role of the subtropical jet stream during heat wave events over north-central Greece, Meteorol. Atmos. Phys., 94, 219–233, 2006.
Caldeira, M. C., Lecomte, X., David, T. S., Pinto, J. G., Bugalho, M. N., and Werner, C.: Synergy of extreme drought and plant invasion reduce ecosystem functioning and resilience, Sci. Rep.-UK, 5, 15110, https://doi.org/10.1038/srep15110, 2015.
Caloiero, T., Veltri, S., Caloiero, P., and Frustaci, F.: Drought analysis in Europe and in the Mediterranean basin using the standardized precipitation index, Water, 10, 1043, https://doi.org/10.3390/w10081043, 2018.
Catto, J. L. and Dowdy, A.: Understanding compound hazards from a weather system perspective, Weather and Climate Extremes, 32, 100313, https://doi.org/10.1016/j.wace.2021.100313, 2021.
Cavicchia, L., von Storch, H., and Gualdi, S.: Mediterranean tropical-like cyclones in present and future climate, J. Climate, 27, 7493–7501, 2014.
Charlton-Perez, A. J., Aldridge, R. W., Grams, C. M., and Lee, R.: Winter pressures on the UK health system dominated by the Greenland blocking weather regime, Weather and Climate Extremes, 25, 100218, https://doi.org/10.1016/j.wace.2019.100218, 2019.
Chenoweth, J., Hadjinicolaou, P., Bruggeman, A., Lelieveld, J., Levin, Z., Lange, M. A., Xoplaki, E., and Hadjikakou, M.: Impact of climate change on the water resources of the eastern Mediterranean and Middle East region: Modeled 21st century changes and implications, Water Resour. Res., 47, W06506, https://doi.org/10.1029/2010WR010269, 2011.
Cherif, S., Doblas-Miranda, E., Lionello, P., Borrego, C., Giorgi, F., Iglesias, A., Jebari, S., Mahmoudi, E., Moriondo, M., Pringault, O., Rilov, G., Somot S., Tsikliras, A., Vila, M., and Zittis, G.: Drivers of change, in: Climate and Environmental Change in the Mediterranean Basin – Current Situation and Risks for the Future. First Mediterranean Assessment Report, edited by: Cramer, W., Guiot, J., and Marini, K., Union for the Mediterranean, Plan Bleu, UNEP/MAP, Marseille, France, 128 pp., https://www.medecc.org/wp-content/uploads/2020/11/MedECC_MAR1_2_Drivers.pdf (last access: 5 April 2022), in press, 2020.
Chronis, T., Papadopoulos, V., and Nikolopoulos, E. I.: QuickSCAT observations of extreme wind events over the Mediterranean and Black Seas during 2000–2008, Int. J. Climatol., 31, 2068–2077, 2011.
Cid, A., Menéndez, M., Castanedo, S., Abascal, A. J., Méndez, F. J., and Medina, R.: Long-term changes in the frequency, intensity and duration of extreme storm surge events in southern Europe, Clim. Dynam., 46, 1503–1516, 2016.
Çolak, E. and Sunar, F.: Evaluation of forest fire risk in the Mediterranean Turkish forests: A case study of Menderes region, Izmir, Int. J. Disast. Risk Re., 45, 101479, https://doi.org/10.1016/j.ijdrr.2020.101479, 2020.
Collier, C. G.: Flash flood forecasting: What are the limits of predictability?, Q. J. Roy. Meteor. Soc., 133, 3–23, https://doi.org/10.1002/qj.29, 2007.
Constantinidou, K., Hadjinicolaou, P., Zittis, G., and Lelieveld, J.: Effects of climate change on the yield of winter wheat in the eastern Mediterranean and Middle East, Clim. Res., 69, 129–141, 2016.
Conte, D. and Lionello, P.: Characteristics of large positive and negative surges in the Mediterranean Sea and their attenuation in future climate scenarios, Global Planet. Change, 111, 159–173, https://doi.org/10.1016/j.gloplacha.2013.09.006, 2013.
Cook, B. I., Smerdon, J. E., Seager, R., and Coats, S.: Global warming and 21st century drying, Clim. Dynam., 43, 2607–2627, 2014.
Cook, B. I., Anchukaitis, K. J., Touchan, R., Meko, D. M., and Cook, E. R.: Spatiotemporal drought variability in the Mediterranean over the last 900 years, J. Geophys. Res., 121, 2060–2074, https://doi.org/10.1002/2015jd023929, 2016.
Coppola, E., Sobolowski, S., Pichelli, E., Raffaele, F., Ahrens, B., Anders, I., Ban, N., Bastin, S., Belda, M., Belusic, D., Caldas-Alvarez, A., Cardoso, R. M., Davolio, S., Dobler, A., Fernandez, J., Fita, L., Fumiere, Q., Giorgi, F., Goergen, K., Güttler, I., Halenka, T., Heinzeller, D., Hodnebrog, Q., Jacob, D., Kartsios, S., Katragkou, E., Kendon, E., Khodayar, S., Kunstmann, H., Knist, S., Lavín-Gullón, A., Lind, P., Lorenz, T., Maraun, D., Marelle, L., van Meijgaard, E., Milovac, J., Myhre, G., Panitz, H. J., Piazza, M., Raffa, M., Raub, T., Rockel, B., Schär, C., Sieck, K., Soares, P. M. M., Somot, S., Srnec, L., Stocchi, P., Tölle, M. H., Truhetz, H., Vautard, R., de Vries, H., and Warrach-Sagi, K.: A first-of-its-kind multi-model convection permitting ensemble for investigating convective phenomena over Europe and the Mediterranean, Clim. Dynam., 43, 3–34, https://doi.org/10.1007/s00382-018-4521-8, 2020.
Cramer, W., Guiot, J., Fader, M., Garrabou, J., Gattuso, J. P., Iglesias, A., Lange, M. A., Lionello, P., Llasat, M. C., Paz, S., and Penuelas, J.: Climate change and interconnected risks to sustainable development in the Mediterranean, Nat. Clim. Change, 8, 972–980, 2018.
Crausbay, S. D., Ramirez, A. R., Carter, S. L., Cross, M. S., Hall, K. R., Bathke, D. J., Betancourt, J. L., Colt, S., Cravens, A. E., Dalton, M. S., Dunham, J. B., Hay, L. E., Hayes, M. J., McEvoy, J., McNutt, C. A., Moritz, M. A., Nislow, K. H., Raheem, N., and Sanford, T.: Defining ecological drought for the twenty-first century, B. Am. Meteorol. Soc., 98, 2543–2550, 2017.
Dafka, S., Toreti, A., Zanis, P., Xoplaki, E., and Luterbacher, J.: Twenty-first-century changes in the Eastern Mediterranean Etesians and associated midlatitude atmospheric circulation, J. Geophys. Res.-Atmos., 124, 12741–12754, 2019.
Daliakopoulos, I. N., Panagea, I. S., Tsanis, I. K., Grillakis, M. G., Koutroulis, A. G., Hessel, R., Mayor, A. G., and Ritsema, C. J.: Yield response of Mediterranean rangelands under a changing climate, Land. Degrad. Dev., 28, 1962–1972, 2017.
David-Novak, H. B., Morin, E., and Enzel, Y.: Modern extreme storms and the rainfall thresholds for initiating debris flows on the hyperarid western escarpment of the Dead Sea Israel, GSA Bulletin, 116, 718–728, https://doi.org/10.1130/B25403.2, 2004.
Dayan, U. and Abramski, R.: Heavy rain in the Middle East related to unusual jet stream properties, B. Am. Meteorol. Soc., 64, 1138–1140, https://doi.org/10.1175/1520-0477(1983)064<1138:HRITME>2.0.CO;2, 1983.
Dayan, U. and Morin, E.: Flash flood-producing rainstorms over the Dead Sea, Geol. Soc. Am., 401, 53–62, https://doi.org/10.1130/2006.2401(04), 2006.
Dayan, U., Ziv, B., Margalit, A., Morin, E., and Sharon, D.: A severe autumn storm over the Middle-East: Synoptic and mesoscale convection analysis, Theor. Appl. Climatol., 69, 103–122, https://doi.org/10.1007/s007040170038, 2001.
Dayan, U., Nissen, K., and Ulbrich, U.: Review Article: Atmospheric conditions inducing extreme precipitation over the eastern and western Mediterranean, Nat. Hazards Earth Syst. Sci., 15, 2525–2544, https://doi.org/10.5194/nhess-15-2525-2015, 2015.
de Vries, A. J., Tyrlis, E., Edry, D., Krichak, S. O., Steil, B., and Lelieveld, J.: Extreme precipitation events in the Middle East: dynamics of the active Red Sea trough, J. Geophys. Res.-Atmos., 118, 7087–7108, https://doi.org/10.1002/jgrd.50569, 2013.
De Vries, A. J., Ouwersloot, H. G., Feldstein, S. B., Riemer, M., El Kenawy, A. M., McCabe, M. F., and, Lelieveld, J.: Identification of tropical-extratropical interactions and extreme precipitation events in the Middle East based on potential vorticity and moisture transport, J. Geophys. Res.-Atmos., 123, 861–881, https://doi.org/10.1002/2017JD027587, 2018.
Deryng, D., Conway, D., Ramankutty, N., Price, J., and Warren, R.: Global crop yield response to extreme heat stress under multiple climate change futures, Environ. Res. Lett., 9, 034011, https://doi.org/10.1088/1748-9326/9/3/034011, 2014.
Diakakis, M., Deligiannakis, G., Katsetsiadou, K., Antoniadis, Z., and Melaki, M.: Mapping and classification of direct flood impacts in the complex conditions of an urban environment, The case study of the 2014 flood in Athens, Greece, Urban Water J., 14, 1065–1074, https://doi.org/10.1080/1573062X.2017.1363247, 2017.
Diakakis, M., Andreadakis, E., Nikolopoulos, E. I., Spyrou, N. I., Gogou, M. E., Deligiannakis, G., Katsetsiadou, N. K., Antoniadis, Z., Melaki, M., Georgakopoulos, A., Tsaprouni, K., Kalogiros, J., and Lekkas, E.: An integrated approach of ground and aerial observations in flash flood disaster investigations: The case of the 2017 Mandra flash flood in Greece, Int. J. Disaster Risk Re,, 33, 290–309, 2019.
Diakakis, M., Damigos, D. G., and Kallioras, A.: Identification of patterns and influential factors on civil protection personnel opinions and views on different aspects of flood risk management: The case of Greece, Sustainability, 12, 5585, https://doi.org/10.3390/su12145585, 2020.
Diffenbaugh, N. S., Pal, J. S., Giorgi, F., and Gao, X.: Heat stress intensification in the Mediterranean climate change hotspot, Geophys. Res. Lett., 34, L11706, https://doi.org/10.1029/2007GL030000, 2007.
Donat, M. G., Peterson, T. C., Brunet, M., King, A. D., Almazroui, M., Kolli, R. K., Boucherf, D., Al-Mulla, A. Y., Nour, A. Y., Aly, A. A., Nada, T. A. A., Semawi, M. M., Al Dashti, H. A., Salhab, T. G., El Fadli, K. I., Muftah, M. K., Dah Eida, S., Badi, W., Driouech, F., El Rhaz, K., Abubaker, M. J. Y., Ghulam, A. S., Erayah, A. S., Mansour, M. B., Alabdouli, W. O., Al Dhanhani, J. S., and Al Shekaili, M. N.: Changes in extreme temperature and precipitation in the Arab region: Long-term trends and variability related to ENSO and NAO, Int. J. Climatol., 34, 581–592, 2014.
Driouech, F., Elrhaz, K., Moufouma, W., Khadija, O., and Saloua, A.: Assessing future changes of climate extreme events in the CORDEX – MENA region using regional climate model ALADIN, Earth Syst. Environ., 4, 477–492, https://doi.org/10.1007/s41748-020-00169-3, 2020.
Drobinski, P., Alpert, P., Cavicchia, L., Flaounas, E., Hochman, A., and Kotroni, V.: Strong winds: observed trends, future projections, Sub-chap. 1.3.2, in: The Mediterranean region under climate change: a scientific update, IRD éditions, https://books.openedition.org/irdeditions/23139?lang=de#authors (last access: 5 April 2022), 2018.
Dubrovský, M., Hayes, M., Duce, P., Trnka, M., Svoboda, M., and Zara, P.: Multi-GCM projections of future drought and climate variability indicators for the Mediterranean region, Reg. Environ. Change, 14, 1907–1919, 2014.
Dupuy, J. L., Fargeon, H., Martin-StPaul, N., Pimont, F., Ruffault, J., Guijarro, M., Hernando, C., Madrigal, J., and Fernandes, P.: Climate change impact on future wildfire danger and activity in southern Europe: a review, Ann. Forest Sci., 77, 35, https://doi.org/10.1007/s13595-020-00933-5, 2020.
Emanuel, K. A.: An air-sea interaction theory for tropical cyclones. Part I: Steady-state maintenance, J. Atmos. Sci., 43, 585–604, 1986.
Enzel, Y., Bookman (Ken Tor), R., Sharon, D., Gvirtzman, H., Dayan, U., Ziv, B., and Stein, M.: Late Holocene climates of the Near East deduced from Dead Sea level variations and modern regional winter rainfall, Quaternary Res., 60, 263–273, https://doi.org/10.1016/j.yqres.2003.07.011, 2003.
Farhan, Y. and Anbar, A.: Fragile landscape: Impact and consequences of May 2014 flash-flood disaster in the Aqaba area, Southern Jordan, Research Journal of Environmental and Earth Sciences, 6, 451–465, 2014.
Fathy, I., Abd-Elhamid, H. F., and Negm, A. M.: Prediction and mitigation of flash floods in Egypt, in: Flash Floods in Egypt, Advances in Science, Technology & Innovation (IEREK Interdisciplinary Series for Sustainable Development), edited by: Negm, A., Springer, Cham, https://doi.org/10.1007/978-3-030-29635-3_15, 2020.
Fatichi, S., Ivanov, V. Y., Paschalis, A., Peleg, N., Molnar, P., Rimkus, S., Kim, J., Burlando, P., and Caporali, E.: Uncertainty partition challenges the predictability of vital details of climate change, Earth's Future, 4, 240–251, 2016.
Ferrarezi, R. S., Rodriguez, K., and Sharp, D.: How historical trends in Florida all-citrus production correlate with devastating hurricane and freeze events, Weather, 75, 77–83, https://doi.org/10.1002/wea.3512, 2019.
Fink, A. H., Brücher, T., Krüger, A., Leckebusch, G. C., Pinto, J. G., and Ulbrich, U.: The 2003 European summer heatwaves and drought-synoptic diagnosis and impacts, Weather, 59, 209–216, 2004.
Finnè, M., Holmgren, K., Sundqvist, H. S., Weiberg, E., and Lindblom, M.: Climate in the eastern Mediterranean, and adjacent regions, during the past 6000 years – A review, J. Archaeol. Sci., 38, 3153–3173, https://doi.org/10.1016/j.jas.2011.05.007, 2011.
Fischer, E. M., Seneviratne, S. I., Lüthi, D., and Schär, C.: Contribution of land-atmosphere coupling to recent European summer heat waves, Geophys. Res. Lett., 34, L06707, https://doi.org/10.1029/2006GL029068, 2007.
Fita, L. and Flaounas, E.: Medicanes as subtropical cyclones: The December 2005 case from the perspective of surface pressure tendency diagnostics and atmospheric water budget, Q. J. Roy. Meteor. Soc., 144, 1028–1044, 2018.
Flaounas, E., Raveh-Rubin, S., Wernli, H., Drobinski, P., and Bastin, S.: The dynamical structure of intense Mediterranean cyclones, Clim. Dynam., 44, 2411–2427, 2015.
Flaounas, E., Kelemen, F. D., Wernli, H., Gaertner, M. A., Reale, M., Sanchez-Gomez, E., Lionello, P., Calmanti, S., Podrascanin, Z., Somot, S., Akhtar, N., Romera, R., and Conte, D.: Assessment of an ensemble of ocean–atmosphere coupled and uncoupled regional climate models to reproduce the climatology of Mediterranean cyclones, Clim. Dynam., 51, 1023–1040, 2018a.
Flaounas, E., Kotroni, V., Lagouvardos, K., Gray, S. L., Rysman, J. F., and Claud, C.: Heavy rainfall in Mediterranean cyclones. Part I: contribution of deep convection and warm conveyor belt, Clim. Dynam., 50, 2935–2949, 2018b.
Flaounas, E., Davolio, S., Raveh-Rubin, S., Pantillon, F., Miglietta, M. M., Gaertner, M. A., Hatzaki, M., Homar, V., Khodayar, S., Korres, G., Kotroni, V., Kushta, J., Reale, M., and Ricard, D.: Mediterranean cyclones: current knowledge and open questions on dynamics, prediction, climatology and impacts, Weather Clim. Dynam., 3, 173–208, https://doi.org/10.5194/wcd-3-173-2022, 2022.
Funatsu, B. M., Claud, C., and Chaboureau, J. P.: Comparison between the large-scale environments of moderate and intense precipitating systems in the Mediterranean region, Mon. Weather Rev. 137, 3933–3959, 2009.
Garfinkel, C. I., Adam, O., Morin, E., Enzel, Y., Elbaum, E., Bartov, M., Rostkier-Edelstein, D., and Dayan, U.: The role of zonally averaged climate change in contributing to intermodel spread in CMIP5 predicted local precipitation changes, J. Climate, 33, 1141–1154, 2020.
Georgiou, G. K., Christoudias, T., Proestos, Y., Kushta, J., Hadjinicolaou, P., and Lelieveld, J.: Air quality modelling in the summer over the eastern Mediterranean using WRF-Chem: chemistry and aerosol mechanism intercomparison, Atmos. Chem. Phys., 18, 1555–1571, https://doi.org/10.5194/acp-18-1555-2018, 2018.
Giles, B. D., Balafoutis, C., and Maheras, P.: Too hot for comfort: the heatwaves in Greece in 1987 and 1988, Int. J. Biometeorol., 34, 98–104, 1990.
Giorgi, F.: Climate change Hot-spots, Geophys. Res. Lett., 33, L08707, https://doi.org/10.1029/2006GL025734, 2006.
Givati, A., Thirel, G., Rosenfeld, D., and Paz, D.: Climate change impacts on streamflow at the upper Jordan river based on an ensemble of regional climate models, J. Hydrol. Reg. Stud., 21, 92–109, 2019.
Gleick, P. H.: Water, drought, climate change, and conflict in Syria, Weather. Clim. Soc., 6, 331–340, 2014.
González-Alemán, J. J., Pascale, S., Gutierrez-Fernandez, J., Murakami, H., Gaertner, M. A., and Vecchi, G. A.: Potential increase in hazard from Mediterranean hurricane activity with global warming, Geophys. Res. Lett., 46, 1754–1764, https://doi.org/10.1029/2018GL081253, 2019.
Gosling, S. N., Lowe, J. A., McGregor, G. R., Pelling, M., and Malamud, B. D.: Associations between elevated atmospheric temperature and human mortality: A critical review of the literature, Climatic Change, 92, 299–341, 2009.
Grimm, N. B., Faeth, S. H., Golubiewski, N. E., Redman, C. L., Jianguo, W., Xuemei, B., and Briggs, J. M.: Global change and the ecology of cities, Science, 319, 756–760, 2008.
Grodek, T., Jacoby, Y., Morin, E., and Katz, O.: Effectiveness of exceptional rainstorms on a small Mediterranean basin, Geomorphology, 159–160, 156–168, https://doi.org/10.1016/j.geomorph.2012.03.016, 2012.
Güner Bacanli, Ü.: Trend analysis of precipitation and drought in the Aegean region, Turkey, Meteorol. Appl., 24, 239–249, https://doi.org/10.1002/met.1622, 2017.
Harris, I., Osborn, T. J., Jones, P. and Lister, D.: Version 4 of the CRU TS monthly high-resolution gridded multivariate climate dataset, Sci. Data, 7, 109, https://doi.org/10.1038/s41597-020-0453-3, 2020 (data available at: https://crudata.uea.ac.uk/cru/data/hrg/cru_ts_4.04/, last access: 5 April 2022).
Hersbach, H., Bell, B., Berrisford, P., Hirahara, S., Horányi, A., Muñoz-Sabater, J., Nicolas, J., Peubey, C., Radu, R., Schepers, D., Simmons, A., Soci, C., Abdalla, S., Abellan, X., Balsamo, G., Bechtold, P., Biavati, G., Bidlot, J., Bonavita, M., De Chiara, G., Dick Dee, P., Diamantakis, M., Dragani, R., Flemming, J., Forbes, R., Fuentes, M., Geer, A. Haimberger, L., Healy, S., Hogan, R. J., Hólm, E., Janisková, M., Keeley, S., Laloyaux, P., Lopez, P., Lupu, C., Radnoti, G., de Rosnay, P., Rozum, I., Vamborg, F., Villaume, S., and Thépaut, J. N.: The ERA5 global reanalysis, Q. J. Roy. Meteor. Soc., 146, 1999–2049, https://doi.org/10.1002/qj.3803, 2020 (data available at: https://www.ecmwf.int/en/forecasts/datasets/reanalysis-datasets/era5, last access: 5 April 2022).
Hochman, A., Harpaz, T., Saaroni, H., and Alpert, P.: Synoptic classification in 21st century CMIP5 predictions over the Eastern Mediterranean with focus on cyclones, Int. J. Climatol., 38, 1476–1483, https://doi.org/10.1002/joc.5260, 2018a.
Hochman, A., Harpaz, T., Saaroni, H., and Alpert, P.: The seasons' length in 21st century CMIP5 projections over the eastern Mediterranean, Int. J. Climatol., 38, 2627–2637, 2018b.
Hochman, A., Mercogliano, P., Alpert, P., Saaroni, H., and Bucchignani, E.: High-resolution projection of climate change and extremity over Israel using COSMO-CLM, Int. J. Climatol., 38, 5095–5106, https://doi.org/10.1002/joc.5714, 2018c.
Hochman, A., Alpert, P., Harpaz, T., Saaroni, H., and Messori, G.: A new dynamical systems perspective on atmospheric predictability: Eastern Mediterranean weather regimes as a case study, Sci. Adv., 5, eaau0936, https://doi.org/10.1126/sciadv.aau0936, 2019a.
Hochman, A., Kunin, P., Alpert, P., Harpaz, T., Saaroni, H., and Rostkier-Edelstein, D.: Weather regimes and analogues downscaling of seasonal precipitation for the 21st century; A case study over Israel, Int. J. Climatol., 40, 2062–2077, https://doi.org/10.1002/joc.6318, 2019b.
Hochman, A., Alpert, P., Baldi, P., Bucchignani, E., Coppola, E., Dahdal, Y., Davidovitch, N., Georgiades, P., Helgert, S., Khreis, H., Levine, H., Materia, S., Negev, M., Salah, I., Shaheen, M., and Giorgi, F.: Inter-disciplinary regional collaboration for public health adaptation to climate change in the eastern Mediterranean, B. Am. Meteorol. Soc., 101, E1685–E1689, https://doi.org/10.1175/BAMS-D-20-0065.1, 2020a.
Hochman, A., Alpert, P., Kunin, P., Rostkier-Edelstein, D., Harpaz, T., Saaroni, H., and Messori, G.: The dynamics of cyclones in the twentyfirst century: The Eastern Mediterranean as an example, Clim. Dynam., 54, 561–574, https://doi.org/10.1007/s00382-019-05017-3, 2020b.
Hochman, A., Alpert, P., Negev, M., Abdeen, Z., Mohsen Abdeen, A., Pinto, J. G., and Levine, H.: The relationship between cyclonic weather regimes and seasonal Influenza over the eastern Mediterranean, Sci. Total Environ., 750, 141686, https://doi.org/10.1016/j.scitotenv.2020.141686, 2020c.
Hochman, A., Scher, S., Quinting, J., Pinto, J. G., and Messori, G.: Dynamics and predictability of cold spells over the Eastern Mediterranean, Clim. Dynam., 1–18, https://doi.org/10.1007/s00382-020-05465-2, 2020d.
Hochman, A., Scher, S., Quinting, J., Pinto, J. G., and Messori, G.: A new view of heat wave dynamics and predictability over the eastern Mediterranean, Earth Syst. Dynam., 12, 133–149, https://doi.org/10.5194/esd-12-133-2021, 2021a.
Hochman, A., Rostkier-Edelstein, D., Kunin, P., and Pinto, J. G.: Changes in the characteristics of “wet” and “dry” Red Sea Trough over the Eastern Mediterranean in CMIP5 climate projections, Theor. Appl. Climatol., 143, 781–794, https://doi.org/10.1007/s00704-020-03449-0, 2021b.
Hoerling, M., Eischeid, J., Perlwitz, J., Quan, X., Zhang, T., and Pegion, P.: On the increased frequency of Mediterranean drought, J. Climate, 25, 2146–2161, https://doi.org/10.1175/JCLI-D-11-00296.1, 2012.
Hosseini, S. R., Scaioni, M., and Marani, M.: Extreme Atlantic hurricane probability of occurrence through the Metastatistical Extreme Value Distribution. Geophy. Res. Lett., 47, 2019GL086138, https://doi.org/10.1029/2019GL086138, 2020.
Houssos, E. E., Lolis, C. J., and Bartzokas, A.: The atmospheric conditions over Europe and the Mediterranean, favoring snow events in Athens, Greece, Adv. Geosci., 12, 127–135, https://doi.org/10.5194/adgeo-12-127-2007, 2007.
Hueging, H., Haas, R., Born, K., Jacob, D., and Pinto, J. G.: Regional changes in wind energy potential over Europe using regional climate model ensemble projections, J. Appl. Meteorol. Clim., 52, 903–917, 2013.
Inbar, M. and Bruins, H. J.: Environmental impact of multi-annual drought in the Jordan Kinneret watershed, Israel, Land. Degrad. Dev., 15, 243–256, https://doi.org/10.1002/ldr.612, 2004.
Inbar, M., Tamir, M., and Wittenberg, L.: Runoff and erosion processes after a forest fire in Mount Carmel, a Mediterranean area, Geomorphology, 24, 17–33, 1998.
IPCC: Climate Change 2021: The Physical Science Basis, Contribution of Working Group I to the Sixth Assessment Report of the Intergovernmental Panel on Climate Change, edited by: Masson-Delmotte, V., Zhai, P., Pirani, A., Connors, S. L., Péan, C., Berger, S., Caud, N., Chen, Y., Goldfarb, L., Gomis, M. I., Huang, M., Leitzell, K., Lonnoy, E., Matthews, J. B. R., Maycock, T. K., Waterfield, T., Yelekçi, O., Yu, R., and Zhou, B., Cambridge University Press, https://www.ipcc.ch/report/ar6/wg1/, (last access: 5 April 2022), in press, 2021.
Ismail, N., Iskander, M., and El-Sayed, W.: Assessment of coastal flooding at southern Mediterranean with global outlook for lowland coastal zones, Coastal Engineering Proceedings, 1, structures.83, https://doi.org/10.9753/icce.v33.structures.83, 2012.
Israel Meteorological Service: Summary of the 10–14 December, 2013 weather event, https://ims.gov.il/sites/default/files/2020-09/sumdec10_14_2013.pdf (last access: 26 December 2021), 2013 (in Hebrew).
Jacob, D., Petersen, J., Eggert, B., Alias, A., Christensen, O. B., Bouwer, L. M., Braun, A., Colette, A., Déqué, M., Georgievski, G., Georgopoulou, E., Gobiet, A., Menut, L., Nikulin, G., Haensler, A., Hempelmann, N., Jones, C., Keuler, K., Kovats, S., Kröner, N., Kotlarski, S., Kriegsmann, A., Martin, E., van Meijgaard, E., Moseley, C., Pfeifer, S., Preuschmann, S., Radermacher, C., Radtke, K., Rechid, D., Rounsevell, M., Samuelsson, P., Somot, S., Soussana, J. F., Teichmann, C., Valentini, R., Vautard, R., Weber, B., and Yiou, P.: EURO-CORDEX: New high-resolution climate change projections for European impact research, Reg. Environ. Chang., 14, 563–578, https://doi.org/10.1007/s10113-013-0499-2, 2014.
Jacovides, C. P., Theophilou, C., Tymvios, F. S., and Pashiardes, S.: Wind statistics for coastal stations in Cyprus, Theor. Appl. Climatol., 72, 259–263, 2002.
Jaeger, E. B. and Seneviratne, S. I.: Impact of soil moisture–atmosphere coupling on European climate extremes and trends in a regional climate model, Clim. Dynam., 36, 1919–1939, 2011.
Kahana, R., Ziv, B. Enzel, Y., and Dayan, U.: Synoptic climatology of major floods in the Negev Desert, Israel, Int. J. Climatol., 22, 867–882, https://doi.org/10.1002/joc.766, 2002.
Karali, A., Hatzaki, M., Giannakopoulos, C., Roussos, A., Xanthopoulos, G., and Tenentes, V.: Sensitivity and evaluation of current fire risk and future projections due to climate change: the case study of Greece, Nat. Hazards Earth Syst. Sci., 14, 143–153, https://doi.org/10.5194/nhess-14-143-2014, 2014.
Kelley, C., Ting, M., Seager, R., and Kushnir, Y.: Mediterranean precipitation climatology, seasonal cycle, and trend as simulated by CMIP5, Geophys. Res. Lett., 39, L21703, https://doi.org/10.1029/2012GL053416, 2012.
Kelley, C. P., Mohtadi, S., Cane, M. A., Seager, R., and Kushnir, Y.: Climate change in the Fertile Crescent and implications of the recent Syrian drought, P. Natl. Acad. Sci. USA, 112, 3241–3246, https://doi.org/10.1073/pnas.1421533112, 2015.
Khader, Y. S., Abdelrahman, M., Abdo, N., Al-Sharif, M., Elbetieha, A., Bakir, H., and Alemam, R.: Climate change and health in the Eastern Mediterranean countries: a systematic review, Rev. Environ. Health, 30, 163–181, https://doi.org/10.1515/reveh-2015-0013, 2015.
Kioutsioukis, I., Melas, D., and Zerefos, C.: Statistical assessment of changes in climate extremes over Greece (1955–2002), Int. J. Climatol., 30, 1723-1737, 2010.
Klawa, M. and Ulbrich, U.: A model for the estimation of storm losses and the identification of severe winter storms in Germany, Nat. Hazards Earth Syst. Sci., 3, 725–732, https://doi.org/10.5194/nhess-3-725-2003, 2003.
Klein Tank, A. M. G., Zwiers, F. W., and Zhang, X.: Guidelines on analysis of extremes in a changing climate in support of informed decisions for adaptation, Climate data and monitoring, Geneva, World Meterological Organization, WCDMP-No. 72, WMO-TD No. 1500, https://www.ecad.eu/documents/WCDMP_72_TD_1500_en_1.pdf (last access: 5 April 2022), 2009.
Kodra, E., Steinhaeuser, K., and Ganguly, A. R.: Persisting cold extremes under 21st-century warming scenarios, Geophys. Res. Lett., 38, L08705, https://doi.org/10.1029/2011gl047103, 2011.
Kostopoulou, E. and Jones, P. D.: Assessment of climate extremes in the Eastern Mediterranean, Meteorol. Atmos. Phys., 89, 69–85, 2005.
Kostopoulou, E. and Jones, P. D.: Comprehensive analysis of the climate variability in the eastern Mediterranean, Part II: relationships between atmospheric circulation patterns and surface climatic elements, Int. J. Climatol., 27, 1351–1371, 2007.
Kostopoulou, E., Giannakopoulos, C., Hatzaki, M., Karali, A., Hadjinicolaou, P., Lelieveld, J., and Lange, M. A.: Spatio-temporal patterns of recent and future climate extremes in the eastern Mediterranean and Middle East region, Nat. Hazards Earth Syst. Sci., 14, 1565–1577, https://doi.org/10.5194/nhess-14-1565-2014, 2014.
Koutroulis, A. G. and Tsanis, I. K.: A method for estimating flash flood peak discharge in a poorly gauged basin: Case study for the 13–14 January 1994 flood, Giofiros basin, Crete, Greece, J. Hydrol., 385, 150–164, 2010.
Koutsoyiannis, D. and Baloutsos, G.: Analysis of a long record of annual maximum rainfall in Athens, Greece, and design rainfall inferences, Nat. Hazard, 22, 29-48, 2000.
Koutsoyiannis, D., Kozonis, D., and Manetas, A.: A mathematical framework for studying rainfall intensity-duration-frequency relationships, J. Hydrol., 206, 118–135, 1998.
Krichak, S. O., Alpert, P., and Krishnamurti, T. N.: Interaction of topography and tropospheric flow – A possible generator for the Red Sea Trough?, Meteorol. Atmos. Phys., 63, 149–158, https://doi.org/10.1007/BF01027381, 1997a.
Krichak, S. O., Alpert, P., and Krishnamurti, T. N.: Red Sea Trough/cyclone development – Numerical investigation, Meteorol. Atmos. Phys., 63, 159–169, https://doi.org/10.1007/BF01027382, 1997b.
Kuglitsch, F. G., Toreti, A., Xoplaki, E., Della-Marta, P. M., Zerefos, C. S., Türkeş, M., and Luterbacher, J.: Heat wave changes in the eastern Mediterranean since 1960, Geophys. Res. Lett., 37, L04802, https://doi.org/10.1029/2009GL041841, 2010.
Kushnir, Y., Dayan, U., Ziv, B., Morin, E., and Enzel, Y.: Climate of the Levant: Phenomena and mechanisms. Quaternary of the Levant: Environments, Climate Change, and Humans, edited by: Enzel, Y. and Ofer, B.-Y., Cambridge University Press, https://doi.org/10.1017/9781316106754.004, 31–44, 2017.
Lange, M. A.: Impacts of climate change on the eastern Mediterranean and the Middle East and North Africa region and the water-energy nexus, Atmosphere-Basel, 10, 455, https://doi.org/10.3390/atmos10080455, 2019.
Laviola, S., Levizzani, V., Ferraro, R. R., and Beauchamp, J.: Hailstorm detection by satellite microwave radiometers, Remote Sensing, 12, 621, https://doi.org/10.3390/rs12040621, 2020.
Lelieveld, J., Hadjinicolaou, P., Kostopoulou, E., Chenoweth, J., El Maayar, M., Giannakopoulos, C., Hannides, C., Lange, M. A., Tanarhte, M., Tyrlis, E., and Xoplaki, E.: Climate change and impacts in the Eastern Mediterranean and the Middle East, Clim. Change, 114, 667–687, https://doi.org/10.1007/s10584-012-0418-4, 2012.
Lelieveld, J., Proestos, Y., Hadjinicolaou, P., Tanarhte, M., Tyrlis, E., and Zittis, G.: Strongly increasing heat extremes in the Middle East and North Africa (MENA) in the 21st century, Climatic Change, 137, 245–260, https://doi.org/10.1007/s10584-016-1665-6, 2016.
Leone, M., D'Ippoliti, D., De Sario, M., Analitis, A., Menne, B., Katsouyanni, K., De' Donato, F. K., Basagana, X., Ben Salah, A., Casimiro, E., Dörtbudak, Z., Iñiguez, C., Peretz, C., Wolf, T., and Michelozzi, P.: A time series study on the effects of heat on mortality and evaluation of heterogeneity into European and Eastern-Southern Mediterranean cities: results of EU CIRCE project, Environ. Health, 12, 55, https://doi.org/10.1186/1476-069X-12-55, 2013.
Levy, Y., Burg, A., Yechieli, Y., and Gvirtzman, H.: Displacement of springs and changes in groundwater flow regime due to the T extreme drop in adjacent lake levels: The Dead Sea rift, J. Hydrol., 587, 124928, https://doi.org/10.1016/j.jhydrol.2020.124928, 2020.
Lionello, P., Trigo, I. F., Gil, V., Liberato, M. L., Nissen, K. M., Pinto, J. G., Raible, C. C., Reale, M., Tanzarella, A., Trigo, R. M., and Ulbrich, S.: Objective climatology of cyclones in the Mediterranean region: a consensus view among methods with different system identification and tracking criteria, Tellus A, 68, 29391, https://doi.org/10.3402/tellusa.v68.29391, 2016.
Lionello, P., Conte, D., and Reale, M.: The effect of cyclones crossing the Mediterranean region on sea level anomalies on the Mediterranean Sea coast, Nat. Hazards Earth Syst. Sci., 19, 1541–1564, https://doi.org/10.5194/nhess-19-1541-2019, 2019.
Lipton, K., Grumm, R., and Holmes, R.: Forecasting heat wavesusing climatic anomalies, in: 21st conference on weatheranalysis/17th conference on numerical weather prediciton, 1–5 August 2005, http://ams.confex.com/ams/pdfpapers/94498.pdf (last access: 4 April 2022), 2005.
Liu, W., Sun, F., Lim, W. H., Zhang, J., Wang, H., Shiogama, H., and Zhang, Y.: Global drought and severe drought-affected populations in 1.5 and 2 °C warmer worlds, Earth Syst. Dynam., 9, 267–283, https://doi.org/10.5194/esd-9-267-2018, 2018.
Llasat, M. C., Llasat-Botija, M., Prat, M. A., Porcú, F., Price, C., Mugnai, A., Lagouvardos, K., Kotroni, V., Katsanos, D., Michaelides, S., Yair, Y., Savvidou, K., and Nicolaides, K.: High-impact floods and flash floods in Mediterranean countries: the FLASH preliminary database, Adv. Geosci., 23, 47–55, https://doi.org/10.5194/adgeo-23-47-2010, 2010.
Lu, J., Vecchi, G. A., and Reichler, T.: Expansion of the Hadley cell under global warming, Geophys. Res. Lett., 34, L06805, https://doi.org/10.1029/2006GL028443, 2007.
Lu, Y., Bookman, R., Waldmann, N., and Marco, S.: A 45kyr laminae record from the Dead Sea: implications for basin erosion and floods recurrence, Quaternary Sci. Rev., 229, 106143, https://doi.org/10.1016/j.quascirev.2019.106143, 2020.
Lubczyńska, M. J., Christophi, C. A., and Lelieveld, J.: Heat-related cardiovascular mortality risk in Cyprus: a case-crossover study using a distributed lag non-linear model, Environ. Health., 14, 39, https://doi.org/10.1186/s12940-015-0025-8, 2015.
Ludwig, P. and Hochman, A.: Last glacial maximum hydro-climate and cyclone characteristics in the Levant: a regional modelling perspective, Environ. Res. Lett., 17, 014053, https://doi.org/10.1088/1748-9326/ac46ea, 2022.
Maheras, P., Flocas, H., Tolika, K., Anagnostopoulou, C., and Vafiadis, M.: Circulation types and extreme temperature changes in Greece, Clim. Res., 30, 161–174, 2006.
Mandelmilch, M., Ferenz, M., Mandelmilch, N., and Potchter, O.: Urban Spatial Patterns and Heat Exposure in the Mediterranean City of Tel Aviv, Atmosphere, 11, 963, https://doi.org/10.3390/atmos11090963, 2020.
Mariotti, A., Pan, Y., Zeng, N., and Alessandri, A.: Long-term climate change in the Mediterranean region in the midst of decadal variability, Clim. Dynam., 44, 1437–1456, 2015.
Markantonis, V., Reynaud, A., Karabulut, A., El Hajj, R., Altinbilek, D., Awad, I. M., Bruggeman, A., Constantianos, V., Mysiak, J., Lamaddalena, N., Matoussi, M. S., Monteiro, H., Pistocchi, A., Pretato, U., Tahboub, N., Tunçok, I. K., Ünver, O., Van Ek, R., Willaarts, B., Bülent, S., Zakir, T., and Bidoglio, G.: Can the implementation of the Water-Energy-Food nexus support economic growth in the Mediterranean region? The current status and the way forward, Front. Environ. Sci., 7, 1–11, https://doi.org/10.3389/fenvs.2019.00084, 2019.
Marra, F. and Morin, E.: Autocorrelation structure of convective rainfall in semiarid-arid climate derived from high-resolution X-band radar estimates, Atmos. Res., 200, 126–138, https://doi.org/10.1016/j.atmosres.2017.09.020, 2018.
Marra, F., Nikolopoulos, E. I., Anagnostou, E. N., Bárdossy, A., and Morin, E.: Precipitation frequency analysis from remotely sensed datasets: A focused review, J. Hydrol. 574, 699–705, https://doi.org/10.1016/j.jhydrol.2019.04.081, 2019a.
Marra, F., Zoccatelli, D., Armon, M., and Morin, E.: A simplified MEV formulation to model extremes emerging from multiple nonstationary underlying processes, Adv. Water Resour., 127, 280–290, https://doi.org/10.1016/j.advwatres.2019.04.002, 2019b.
Marra, F., Borga, M., and Morin, E.: A unified framework for extreme sub-daily precipitation frequency analyses based on ordinary events, Geophys. Res. Lett., 47, e2020GL090209, https://doi.org/10.1029/2020GL090209, 2020.
Marra, F., Armon, M., Adam, O., Zoccatelli, D., Gazal, O., Garfinkel, C. I., Rostkier-Edelstein, D., Dayan, U., Enzel, Y., and Morin, E.: Towards narrowing uncertainty in future projections of local extreme precipitation, Geophy. Res. Lett., 48, e2020GL091823, https://doi.org/10.1029/2020GL091823, 2021a.
Marra, F., Armon, M., Borga, M., and Morin, E.: Orographic effect on extreme precipitation statistics peaks at hourly time scales, Geophys. Res. Lett., 48, e2020GL091498, https://doi.org/10.1029/2020GL091498, 2021b.
Martius, O., Pfahl, S., and Chevalier, C.: A global quantification of compound precipitation and wind extremes, Geophy. Res. Lett., 43, 7709–7717, 2016.
Mastrantonas, N., Herrera-Lormendez, P., Magnusson, L., Pappenberger, F., and Matschullat, J.: Extreme precipitation events in the Mediterranean: Spatiotemporal characteristics and connection to large-scale atmospheric flow patterns, Int. J. Climatol., 41, 2710–2728, 2021.
Mathbout, S., Lopez-Bustins, J. A., Roye, D., Martin-Vide, J., Bech, J., and Rodrigo, F. S.: Observed changes in daily precipitation extremes at annual timescale over the eastern Mediterranean during 1961–2012, Pure Appl. Geophys., 175, 3875–3890, https://doi.org/10.1007/s00024-017-1695-7, 2018.
Mazza, E., Ulbrich, U., and Klein, R.: The tropical transition of the October 1996 Medicane in the western Mediterranean Sea: A warm seclusion event, Mon. Weather Rev., 145, 2575–2595, 2017.
Mcvicar, T. R., Roderick, M. L., Donohue, R. J., Li, L. T., Van Niel, T. G., Thomas, A., Grieser, J., Jhajharia, D., Himri, Y., Mahowald, N. M., Mescherskaya, A. V., Kruger, A. C., Rehman, S., and Dinpashoh, Y.: Global review and synthesis of trends in observed terrestrial near-surface wind speeds: Implications for evaporation, J. Hydrol., 416–417, 182–205, 2012.
Merkenschlager, C., Hertig, E., and Jacobeit, J.: Non-stationarities in the relationships of heavy precipitation events in the Mediterranean area and the large-scale circulation in the second half of the 20th century, Global Planet. Change, 151, 108–121, 2017.
Merz, B., Kuhlicke, C., Kunz, M., Pittore, M., Babeyko, A., Bresch, D. N., Domeisen, D. I. V., Feser, F., Koszalka, I., Kreibich, H., Pantillon, F., Parolai, S., Pinto, J. G., Punge, H. J., Rivalta, E., Schröter, K., Strehlow, K., Weisse, R., and Wurpts, A.: Impact forecasting to support emergency management of natural hazards, Rev. Geophys., 58, e2020RG000704, https://doi.org/10.1029/2020RG000704, 2020.
Messer, H., Zinevich, A., and Alpert, P.: Environmental monitoring by wireless communication networks, Science, 312, 713, https://doi.org/10.1126/science.1120034, 2006.
Metzger, A., Marra, F., Smith, J. A., and Morin, E.: Flood frequency estimation and uncertainty in arid/semi-arid regions, J. Hydrol., 590, 125254, https://doi.org/10.1016/j.jhydrol.2020.125254, 2020.
Michaelides, S., Karacostas, T., Sánchez, J. L., Retalis, A., Pytharoulis, I., Homar, V., Romero, R., Zanis, P., Giannakopoulos, C., Bühl, J., Ansmann, A., Merino, A., Melcón, P., Lagouvardos, K., Kotroni, V., Bruggeman, A., López-Moreno, J. I., Berthet, C., Katragkou, E., Tymvios, F., Diofantos, Hadjimitsis, G., Mamouri, R.-E., and Nisantzi, A.: Reviews and perspectives of high impact atmospheric processes in the Mediterranean, Atmos. Res., 208, 4–44, https://doi.org/10.1016/j.atmosres.2017.11.022, 2018.
Miglietta, M. M.: Mediterranean tropical-like cyclones (Medicanes), Atmosphere, 10, 206, https://doi.org/10.3390/atmos10040206, 2019.
Miglietta, M. M. and Rotunno, R.: Development Mechanisms for Mediterranean Tropical-Like Cyclones (Medicanes), Q. J. Roy. Meteor. Soc., 145, 1444–1460, https://doi.org/10.1002/qj.3503, 2019.
Miglietta, M. M., Laviola, S., Malvaldi, A., Conte, D., Levizzani, V., and Price, C.: Analysis of tropical-like cyclones over the Mediterranean Sea through a combined modeling and satellite approach, Geophys. Res. Lett., 40, 2400–2405, https://doi.org/10.1002/grl.50432, 2013.
Miniussi, A. and Marani, M.: Estimation of daily rainfall extremes through the metastatistical extreme value distribution: Uncertainty minimization and implications for trend detection, Water Resour. Res., 56, e2019WR026535, https://doi.org/10.1029/2019WR026535, 2020.
Mishra, A. K. and Singh, V. P.: A review of drought concepts, J. Hydrol., 391, 202–216, https://doi.org/10.1016/j.jhydrol.2010.07.012, 2010.
Mömken, J., Reyers, M., Feldmann, H., and Pinto, J. G.: Future changes of wind speed and wind energy potentials in EURO-CORDEX ensemble simulations, J. Geophys. Res.-Atmos., 123, 6373–6389, https://doi.org/10.1029/2018JD028473, 2018.
Morin, E.: To know what we cannot know: Global mapping of minimal detectable absolute trends in annual precipitation, Water Resour. Res., 47, W07505, https://doi.org/10.1029/2010WR009798, 2011.
Morin, E. and Yakir, H.: Hydrological impact and potential flooding of convective rain cells in a semi-arid environment, Hydrolog. Sci. J., 59, 1353–1362, https://doi.org/10.1080/02626667.2013.841315, 2014.
Morin, E., Harats, N., Jacoby, Y., Arbel, S., Getker, M., Arazi, A., Grodek, T., Ziv, B., and Dayan, U.: Studying the extremes: hydrometeorological investigation of a flood-causing rainstorm over Israel, Adv. Geosci., 12, 107–114, https://doi.org/10.5194/adgeo-12-107-2007, 2007.
Morin, E., Jacoby, Y., Navon, S., and Bet-Halachmi, E.: Towards flash-flood prediction in the dry Dead Sea region utilizing radar rainfall information, Adv. Water Resour., 32, 1066–1076, 2009.
Morin, E., Ryb, T., Gavrieli, I., and Enzel, Y.: Mean, variance and trends of Levant precipitation over the past 4500 years from reconstructed Dead Sea lake levels and stochastic modeling, Quaternary Res., 91, 751e767, https://doi.org/10.1017/qua.2018.98, 2019.
Morsy, M., Sayad, T., and Khamees, A. S.: Towards instability index development for heavy rainfall events over Egypt and the Eastern Mediterranean. Meteorol. Atmos. Phys., 132, 255–272, https:/doi.org/10.1007/s00703-019-00686-5, 2020.
Mueller, B. and Seneviratne, S. I.: Hot days induced by precipitation deficits at the global scale, P. Natl. Acad. Sci. USA, 109, 12398–12403, 2012.
Mukherjee, S. and Mishra, A. K.: Increase in compound drought and heatwaves in a warming world, Geophy. Res. Lett., 48, e2020GL090617, https://doi.org/10.1029/2020GL090617, 2021.
Nairn, J. R. and Fawcett, R. G.: Defining heatwaves: heatwave defined as a heat-impact event servicing all community and business sectors in Australia, CAWCR Technical Report No. 060, Centre for Australian Weather and Climate Research, https://www.cawcr.gov.au/technical-reports/CTR_060.pdf (last access: 5 April 2022), 2013.
Nastos, P. T. and Zerefos, C. S.: Decadal changes in extreme daily precipitation in Greece, Adv. Geosci., 16, 55–62, https://doi.org/10.5194/adgeo-16-55-2008, 2008.
Nastos, P. T. and Zerefos, C. S.: Spatial and temporal variability of consecutive dry and wet days in Greece, Atmos. Res., 94, 616–628, 2009.
Nastos, P. T., Politi, N., and Kapsomenakis, J.: Spatial and temporal variability of the Aridity Index in Greece, Atmos. Res., 119, 140–152, https://doi.org/10.1016/j.atmosres.2011.06.017, 2013a.
Nastos, P. T., Kapsomenakis, J., and Douvis, K. C.: Analysis of precipitation extremes based on satellite and high-resolution gridded data set over Mediterranean basin, Atmos. Res., 131, 46–59, 2013b.
Nastos, P. T., Kasavana Papadimou, K., and Mastagouras, I. T.: Mediterranean tropical-like cyclones: Impacts and composite daily means and anomalies of synoptic patterns, Atmos. Res., 208, 156–166, 2018.
Nastos, P. T., Dalezios, N. R., Faraslis, I. N., Mitrakopoulos, K., Blanta, A., Spiliotopoulos, M., Sakellariou, S., Sidiropoulos, P., and Tarquis, A. M.: Review article: Risk management framework of environmental hazards and extremes in Mediterranean ecosystems, Nat. Hazards Earth Syst. Sci., 21, 1935–1954, https://doi.org/10.5194/nhess-21-1935-2021, 2021.
Naumann, G., Russo, S., Formetta, G., Ibarreta, D., Forzieri, G., Girardello, M., and Feyen, L.: Global warming and human impacts of heat and cold extremes in the EU, Publications Office of the European Union, Luxembourg, JRC118540, https://doi.org/10.2760/47878, 2020.
Negev, M., Dahdal, Y., Khreis, H., Hochman, A., Shaheen, M., Jaghabir, M., Alpert, P., Levine, H., and Davidovitch, N.: Regional lessons from the COVID-19 outbreak in the Middle East: from infectious diseases to climate change adaptation, Sci. Total Environ., 768, 144434, https://doi.org/10.1016/j.scitotenv.2020.144434, 2021.
Neu, U., Akperov, M. G., Bellenbaum, N., Benestad, R., Blender, R., Caballero, R., Cocozza, A., Dacre, H. F., Feng, Y., Fraedrich, K., Grieger, J., Gulev, S., Hanley, J., Hewson, T., Inatsu, M., Keay, K., Kew, S. F., Kindem, I., Leckebusch, G. C., Liberato, M. L. R., Lionello, P., Mokhov, I. I., Pinto, J. G., Raible, C. C., Reale, M., Rudeva, I., Schuster, M., Simmonds, I., Sinclair, M., Sprenger, M., Tilinina, N. D., Trigo, I. F., Ulbrich, S., Ulbrich, U., Wang, X. L., and Wernli, H.: IMILAST: A community effort to intercompare extratropical cyclone detection and tracking algorithms, B. Am. Meteorol. Soc., 94, 529–547, 2013.
Nicault, A., Alleaume, S., Brewer, S., Carrer, M., Nola, P., and Guiot, J.: Mediterranean drought fluctuation during the last 500 years based on tree-ring data, Clim. Dynam., 31, 227–245, 2008.
Nissen, K. M., Leckebusch, G. C., Pinto, J. G., Renggli, D., Ulbrich, S., and Ulbrich, U.: Cyclones causing wind storms in the Mediterranean: characteristics, trends and links to large-scale patterns, Nat. Hazards Earth Syst. Sci., 10, 1379–1391, https://doi.org/10.5194/nhess-10-1379-2010, 2010.
Nissen, K. M., Leckebusch, G. C., Pinto, J. G., and Ulbrich, U.: Mediterranean cyclones and windstorms in a changing climate, Reg. Environ. Change, 14, 1873–1890, 2014.
Ocakoglu, F., Gokceoglu, C., and Ercanoglu, M.: Dynamics of a complex mass movement triggered by heavy rainfall: a case study from NW Turkey, Geomorphology, 42, 329–341, https://doi.org/10.1016/S0169-555X(01)00094-0, 2002.
Osetinsky-Tzidaki, I. and Venger, D.: Extreme Wind Analysis: A Comprehensive Algorithm, in: 100th American Meteorological Society Annual Meeting, AMS, Boston, MA, USA, 12–16 January 2020, 2020.
Palecki, M. A., Changnon, S. A., and Kunkel, K. E.: The nature and impacts of the July 1999 heat wave in the midwestern United States: learning from the lessons of 1995, B. Am. Meteorol. Soc., 82, 1353–1368, 2001.
Papadaskalopoulou, C., Moriondo, M., Lemesios, I., Karali, A., Konsta, A., Dibari, C., Brilli, L., Varotsos, K. V., Stylianou, A., Loizidou, M., Markou, M., and Giannakopoulos, C.: Assessment of total climate change impacts on the agricultural sector of Cyprus, Atmosphere-Basel, 11, 1–17, https://doi.org/10.3390/atmos11060608, 2020.
Papaioannou, G., Kitsara, G., and Athanasatos, S.: Impact of global dimming and brightening on reference evapotranspiration in Greece, J. Geophys. Res.-Atmos., 116, D09107, https://doi.org/10.1029/2010JD015525, 2011.
Pappas, C., Hatzianastassiou, N., and Katsoulis, B. D.: Analysis of cold spells in the Greek region, Clim. Res., 27, 211–223, 2004.
Peleg, N. and Morin, E.: Convective rain cells: Radar-derived spatiotemporal characteristics and synoptic patterns over the eastern Mediterranean, J. Geophys. Res., 117, D15116, https://doi.org/10.1029/2011JD017353, 2012.
Peleg, N., Morin, E., Gvirtzman, H., and Enzel, Y.: Rainfall, spring discharge and past human occupancy in the Eastern Mediterranean, Climatic Change, 112, 769–789, https://doi.org/10.1007/s10584-011-0232-4, 2012.
Peleg, N., Bartov, M., and Morin, E.: CMIP5-predicted climate shifts over the East Mediterranean: implications for the transition region between Mediterranean and semi-arid climates, Int. J. Climatol., 35, 2144–2153, https://doi.org/10.1002/joc.4114, 2015a.
Peleg, N., Shamir, E., Georgakakos, K. P., and Morin, E.: A framework for assessing hydrological regime sensitivity to climate change in a convective rainfall environment: a case study of two medium-sized eastern Mediterranean catchments, Israel, Hydrol. Earth Syst. Sci., 19, 567–581, https://doi.org/10.5194/hess-19-567-2015, 2015b.
Peleg, N., Marra, F., Fatichi, S., Molnar, P., Morin, E., Sharma, A., and Burlando, P.: Intensification of convective rain cells at warmer temperatures observed from high-resolution weather radar data, J. Hydrometeorol., 19, 715–726, https://doi.org/10.1175/JHM-D-17-0158.1, 2018a.
Peleg, N., Marra, F., Fatichi, S., Paschalis, A., Molnar, P., and Burlando, P.: Spatial variability of extreme rainfall at radar subpixel scale, J. Hydrol., 556, 922–933, https://doi.org/10.1016/j.jhydrol.2016.05.033, 2018b.
Perkins-Kirkpatrick S. and Lewis, S. C.: Increasing trends in regional heatwaves, Nat. Commun., 11, 3357, https://doi.org/10.1038/s41467-020-16970-7, 2020.
Petrucci, O., Aceto, L., Bianchi, C., Bigot, V., Brázdil, R., Pereira, S., Kahraman, A., Kılıç, Ö., Kotroni, V., Llasat, M. C., Llasat-Botija, M., Papagiannaki, K., Pasqua, A. A., Řehoř, J., Rossello Geli, J., Salvati, P., Vinet, F., and Zêzere, J. L.: Flood Fatalities in Europe, 1980–2018: Variability, Features, and Lessons to Learn, Water, 11, 1682, https://doi.org/10.3390/w11081682, 2019.
Pfahl, S., Madonna, E., Boettcher, M., Joos, H., and Wernli, H.: Warm conveyor belts in the ERA-Interim dataset (1979–2010). Part II: Moisture origin and relevance for precipitation, J. Climate, 27, 27–40, 2014.
Philandras, C. M., Nastos, P. T., Kapsomenakis, J., Douvis, K. C., Tselioudis, G., and Zerefos, C. S.: Long term precipitation trends and variability within the Mediterranean region, Nat. Hazards Earth Syst. Sci., 11, 3235–3250, https://doi.org/10.5194/nhess-11-3235-2011, 2011.
Pinto, J. G., Ulbrich, U., Leckebusch, G. C., Spangehl, T., Reyers, M., and Zacharias, S.: Changes in storm track and cyclone activity in three SRES ensemble experiments with the ECHAM5/MPI-OM1 GCM, Clim. Dynam., 29, 195–210, 2007.
Pinto, J. G., Karremann, M. K., Born, K., Della-Marta, P. M., and Klawa, M.: Loss potentials associated with European windstorms under future climate conditions, Clim. Res., 54, 1–20, 2012.
Prudhomme, C., Giuntoli, I., Robinson, E. L., Clark, D. B., Arnell, N. W., Dankers, R., Fekete, B. M., Franssen, W., Gerten, D., Gosling, S. N., Hagemann, S., Hannah, D. M., Kim, H., Masaki, Y., Satoh, Y., Stacke, T., Wada, Y., and Wisser, D.: Hydrological droughts in the 21st century, hotspots and uncertainties from a global multimodel ensemble experiment, P. Natl. Acad. Sci. USA, 111, 3262–3267, https://doi.org/10.1073/pnas.1222473110, 2014.
Raveh-Rubin, S. and Wernli, H.: Large-scale wind and precipitation extremes in the Mediterranean: a climatological analysis for 1979–2012, Q. J. Roy. Meteor. Soc., 141, 2404–2417, 2015.
Raveh-Rubin, S. and Wernli, H.: Large-scale wind and precipitation extremes in the Mediterranean: dynamical aspects of five selected cyclone events, Q. J. Roy. Meteor. Soc., 142, 3097–3114, 2016.
Reale, M., David, W., Narvaez, C., Cavicchia, L., Conte, D., Coppola, E., Flaounas, E., Giorgi, F., Gualdi, S., Hochman, A., Li, L., Lionello, P., Podrascanin, Z., Salon, S., Sanchez-Gomez, E., Scoccimarro, E., Sein, D. V., and Somot, S.: Future projections of Mediterranean cyclone characteristics using the Med-CORDEX ensemble of coupled regional climate system models, Clim. Dynam., https://doi.org/10.1007/s00382-021-06018-x, 2021.
Rinat, Y., Marra, F., Zoccatelli, D., and Morin, E.: Controls of flash flood peak discharge in Mediterranean basins and the special role of runoff-contributing areas, J. Hydrol., 565, 846–860, https://doi.org/10.1016/j.jhydrol.2018.08.055, 2018.
Rinat, Y., Marra, F., Armon, M., Metzger, A., Levi, Y., Khain, P., Vadislavsky, E., Rosensaft, M., and Morin, E.: Hydrometeorological analysis and forecasting of a 3 d flash-flood-triggering desert rainstorm, Nat. Hazards Earth Syst. Sci., 21, 917–939, https://doi.org/10.5194/nhess-21-917-2021, 2021.
Romera, R., Gaertner, M. A., Sánchez, E., Domínguez, M., González-Alemán, J. J., and Miglietta, M. M.: Climate change projections of Medicanes with a large multi-model ensemble of regional climate models, Global Planet. Change, 151, 134–143, 2016.
Rubin, S., Ziv, B., and Paldor, N.: Tropical plumes over eastern North Africa as a source of rain in the Middle East, Mon. Weather Rev., 135, 4135–4148, https://doi.org/10.1175/2007MWR1919.1, 2007.
Rummukainen, M.: Changes in climate and weather extremes in the 21st century, WIRES Climate Change, 3, 115–129, https://doi.org/10.1002/wcc.160, 2012.
Ryti, N. R., Guo, Y., and Jaakkola, J. J.: Global association of cold spells and adverse health effects: a systematic review and meta-analysis, Environ. Health Persp., 124, 12–22, https://doi.org/10.1289/ehp.1408104, 2016.
Saaroni, H., Bitan, A., Alpert, P., and Ziv, B.: Continental polar outbreaks into the Levant and eastern Mediterranean, Int. J. Climatol., 16, 1175–1191, 1996.
Saaroni, H., Ziv, B., Bitan, A., and Alpert, P.: Easterly wind storms over Israel, Theor. Appl. Climatol., 59, 61–77, 1998.
Saaroni, H., Ziv, B., Edelson, J., and Alpert, P.: Long-term variations in summer temperatures over the eastern Mediterranean, Geophys. Res. Lett., 30, 1946, https://doi.org/10.1029/2003GL017742, 2003.
Saaroni, H., Halfon, N., Ziv, B., Alpert, P., and Kutiel, H.: Links between the rainfall regime in Israel and location and intensity of Cyprus lows, Int. J. Climatol., 30, 1014–1025, 2010.
Saaroni, H., Harpaz, T., Alpert, P., and Ziv, B.: Automatic identification and classification of the northern part of the Red Sea trough and its application for climatological analysis, Int. J. Climatol., 40, 3607–3622, https://doi.org/10.1002/joc.6416, 2020.
Samuels, R., Rimmer, A., and Alpert, P.: Effect of extreme rainfall events on the water resources of the Jordan River, J. Hydrol., 375, 513–523, 2009.
Samuels, R., Hochman, A., Baharad, A., Givati, A., Levi, Y., Yosef, Y., Saaroni, H., Ziv, B., Harpaz, H., and Alpert, P.: Evaluation and projection of extreme precipitation indices in the eastern Mediterranean based on CMIP5 multi-model ensemble, Int. J. Climatol., 38, 2280–2297, https://doi.org/10.1002/joc.5334, 2018.
Seager, R., Osborn, T. J., Kushnir, Y., Simpson, I. R., Nakamura, J., and Liu, H.: Climate variability and change of mediterranean-type climates, J. Climate, 32, 2887–2915, https://doi.org/10.1175/JCLI-D-18-0472.1, 2019.
Sen, B., Topcu, S., Türkeş, M., Sen, B., and Warner, J. F.: Projecting climate change, drought conditions and crop productivity in Turkey, Clim. Res., 52, 175–191, https://doi.org/10.3354/cr01074, 2012.
Seneviratne, S. I.: Climate science: Historical drought trends revisited, Nature, 491, 338–339, https://doi.org/10.1038/491338a, 2012.
Shata, A. A. and Hanitsch, R.: Evaluation of wind energy potential and electricity generation on the coast of Mediterranean Sea in Egypt, Renew. Energ., 31, 1183–1202, 2006.
Shaw, T. A., Baldwin, M., Barnes, E. A., Caballero, R., Garfinkel, C. I., Hwang, Y. T., Li, C., O'Gorman, P. A., Rivière, G., Simpson, I. R., and Voigt, A.: Storm track processes and the opposing influences of climate change, Nat. Geosci., 9, 656–664, 2016.
Sherwood, S. C. and Huber, M.: An adaptability limit to climate change due to heat stress, P. Natl. Acad. Sci. USA, 107, 9552–9555, 2010.
Shmilovitz, Y., Morin, E., Rinat, Y., Haviv, I., Carmi, G., Mushkin, A., and Enzel, Y.: Linking frequency of rainstorms, runoff genera- tion and sediment transport across hyperarid talus-pediment slopes, Earth Surf. Proc. Land., 49, 1644–1659, 2020.
Shmilovitz, Y., Marra, F., Wei, H., Argaman, E., Nearing, M., Goodrich, D., Assouline, S., and Morin, E.: Frequency analysis of storm-scale soil erosion and characterization of extreme erosive events by linking the DWEPP model and a stochastic rainfall generator, Sci. Total Environ., 787, 147609, https://doi.org/10.1016/j.scitotenv.2021.147609, 2021.
Shohami, D., Dayan, U., and Morin, E.: Warming and drying of the eastern Mediterranean: Additional evidence from trend analysis, J. Geophys. Res., 116, D22101, https://doi.org/10.1029/2011JD016004, 2011.
Sillmann, J., Kharin, V. V., Zwiers, F. W., Zhang, X., and Bronaugh, D.: Climate extremes indices in the CMIP5 multimodel ensemble: Part 2. Future climate projections, J. Geophys. Res.-Atmos., 118, 2473–2493, https://doi.org/10.1002/jgrd.50188, 2013.
Smart, D. J. and Browning, K. A.: Attribution of strong winds to a cold conveyor belt and sting jet, Q. J. Roy. Meteor. Soc., 140, 595–610, 2014.
Solberg, S., Hov, Ø., Isaksen, I. S. A., Coddeville, P., De Backer, H., Forster, C., Orsolini, Y., and Uhse, K.: European surface ozone in the extreme summer 2003, J. Geophys. Res., 113, D07307, https://doi.org/10.1029/2007JD009098, 2008.
Sousa, P. M., Trigo, R. M., Aizpurua, P., Nieto, R., Gimeno, L., and Garcia-Herrera, R.: Trends and extremes of drought indices throughout the 20th century in the Mediterranean, Nat. Hazards Earth Syst. Sci., 11, 33–51, https://doi.org/10.5194/nhess-11-33-2011, 2011.
Spinoni, J., Vogt, J. V., Naumann, G., Barbosa, P., and Dosio, A.: Will drought events become more frequent and severe in Europe?, Int. J. Climatol., 38, 1718–1736, https://doi.org/10.1002/joc.5291, 2018.
Spinoni, J., Barbosa, P., De Jager, A., McCormick, N., Naumann, G., Vogt, J. V., Magni, D., Masante, D., and Mazzeschi, M.: A new global database of meteorological drought events from 1951 to 2016, J. Hydrol. Reg. Stud., 22, 100593, https://doi.org/10.1016/j.ejrh.2019.100593, 2019.
Spinoni, J., Barbosa, P., Bucchignani, E., Cassano, J., Cavazos, T., Christensen, J. H., Christensen, O. B., Coppola, E., Evans, J., Geyer, B., Giorgi, F., Hadjinicolaou, P., Jacob, D., Katzfey, J., Koenigk, T., Laprise, R., Lennard, C. J., Kurnaz, M. L., Li, D., Llopart, M., McCormick, N., Naumann, G., Nikulin, G., Ozturk, T., Panitz, H.-J., Porfirio da Rocha, R., Rockel, B., Solman, S. A., Syktus, J., Tangang, F., Teichmann, C., Vautard, R., Vogt, J. V., Winger, K., Zittis, G., and Dosio, A.: Future Global Meteorological Drought Hot Spots: A Study Based on CORDEX Data, J. Climate, 33, 3635–3661, https://doi.org/10.1175/jcli-d-19-0084.1, 2020.
Spyrou, C., Varlas, G., Pappa, A., Mentzafou, A., Katsafados, P., Papadopoulos, A., Anagnostou, M. N., and Kalogiros, J.: Implementation of a Nowcasting Hydrometeorological System for Studying Flash Flood Events: The Case of Mandra, Greece, Remote Sens., 12, 2784, https://doi.org/10.3390/rs12172784, 2020.
Sui, C.-H., Li, X., and Yang, M.-J.: On the definition of precipitation efficiency, J. Atmos. Sci., 64, 4506–4513, https://doi.org/10.1175/2007JAS2332.1, 2007.
Tabari, H. and Willems, P.: More prolonged droughts by the end of the century in the Middle East, Environ. Res. Lett., 13, 104005, https://doi.org/10.1088/1748-9326/aae09c, 2018.
Tanarhte, M., Hadjinicolaou, P., and Lelieveld, J.: Intercomparison of temperature and precipitation data sets based on observations in the Mediterranean and the Middle East, J. Geophys. Res.-Atmos., 117, D12102, https://doi.org/10.1029/2011JD017293, 2012.
Tarolli, P., Borga, M., Morin, E., and Delrieu, G.: Analysis of flash flood regimes in the North-Western and South-Eastern Mediterranean regions, Nat. Hazards Earth Syst. Sci., 12, 1255–1265, https://doi.org/10.5194/nhess-12-1255-2012, 2012.
Tayanç, M., Karaca, M., and Dalfes, H. N.: March 1987 cyclone (blizzard) over the eastern Mediterranean and Balkan region associated with blocking, Monthly Weather Review, 126, 3036–3047, 1998.
Taylor, K. E. R., Stouffer, J., and Meehl, G. A.: An overview of CMIP5 and the experiment design, B. Am. Meteorol. Soc., 93, 485–498, 2012 (data available at: https://esgf-node.llnl.gov/projects/cmip5/, last access: 5 April 2022).
Tobin, I., Vautard, R., Balog, I., Bréon, F., Jerez, S., Ruti, P. M., Thais, F., Vrac, M., and Yiou, P.: Assessing climate change impacts on European wind energy from ENSEMBLES high-resolution climate projections, Climatic Change, 128, 99–112, https://doi.org/10.1007/s10584-014-1291-0, 2015.
Toreti, A., Xoplaki, E., Maraun, D., Kuglitsch, F. G., Wanner, H., and Luterbacher, J.: Characterisation of extreme winter precipitation in Mediterranean coastal sites and associated anomalous atmospheric circulation patterns, Nat. Hazards Earth Syst. Sci., 10, 1037–1050, https://doi.org/10.5194/nhess-10-1037-2010, 2010.
Toros, H., Kahraman, A., Tilev-Tanriover, S., Geertsema, G., and Cats, G.: Simulating heavy precipitation with HARMONIE, HIRLAM and WRF-ARW: a flash flood case study in Istanbul, Turkey, European J. Sci. Technol., 13, 1–12, 2018.
Touma, D., Ashfaq, M., Nayak, M. A., Kao, S. C., and Diffenbaugh, N. S.: A multi-model and multi-index evaluation of drought characteristics in the 21st century, J. Hydrol., 526, 196–207, https://doi.org/10.1016/j.jhydrol.2014.12.011, 2015.
Tous, M., Zappa, G., Romero, R., Shaffrey, L., and Vidale, P. L.: Projected changes in Medicanes in the HadGEM3 N512 high-resolution global climate model, Clim. Dynam., 47, 1913–1924, 2016.
Tramblay, Y., Koutroulis, A., Samaniego, L., Vicente-Serrano, S. M., Volaire, F., Boone, A., Le Page, M., Llasat, M. C., Albergel, C., Burak, S., Cailleret, M., Kalin, K. C., Davi, H., Dupuy, J. L., Greve, P., Grillakis, M., Hanich, L., Jarlan, L., Martin-StPaul, N., Martínez-Vilalta, J., Mouillot, F., Pulido-Velazquez, D., Quintana-Seguí, P., Renard, D., Turco, M., Türkeş, M., Trigo, R., Vidal, J. P., Vilagrosa, A., Zribi, M., and Polcher, J.: Challenges for drought assessment in the Mediterranean region under future climate scenarios, Earth-Sci. Rev., 210, 103348, https://doi.org/10.1016/j.earscirev.2020.103348, 2020.
Tressol, M., Ordonez, C., Zbinden, R., Brioude, J., Thouret, V., Mari, C., Nedelec, P., Cammas, J.-P., Smit, H., Patz, H.-W., and Volz-Thomas, A.: Air pollution during the 2003 European heat wave as seen by MOZAIC airliners, Atmos. Chem. Phys., 8, 2133–2150, https://doi.org/10.5194/acp-8-2133-2008, 2008.
Tsvieli, Y. and Zangvil, A.: Synoptic climatological analysis of “wet” and “dry” Red Sea troughs over Israel, Int. J. Climatol., 25, 1997–2015, https://doi.org/10.1002/joc.1232, 2005.
Tsvieli, Y. and Zangvil, A.: Synoptic climatological analysis of Red Sea Trough and non-Red Sea Trough rain situations over Israel, Adv. Geosci., 12, 137–143, https://doi.org/10.5194/adgeo-12-137-2007, 2007.
Tubi, A. and Dayan, U.: Tropical Plumes over the Middle East: Climatology and synoptic conditions, Atmos. Res., 145, 168–181, 2014.
Turco, M., Von Hardenberg, J., AghaKouchak, A., Llasat, M. C., Provenzale, A., and Trigo, R. M.: On the key role of droughts in the dynamics of summer fires in Mediterranean Europe, Sci. Rep.-UK, 7, 81, https://doi.org/10.1038/s41598-017-00116-9, 2017a.
Turco, M., Levin, N., Tessler, N., and Saaroni, H.: Recent changes and relations among drought, vegetation and wildfires in the Eastern Mediterranean: The case of Israel, Global Planet. Change, 151, 28–35, https://doi.org/10.1016/j.gloplacha.2016.09.002, 2017b.
Ulbrich, U., Lionello, P., Belusic, D., Jacobeit, J., Knippertz, P., Kuglitsch, F. G., Leckebusch, G. C., Luterbacher, J., Maugeri, M., Maheras, P., and Nissen, K. M.: Climate of the Mediterranean: Synoptic patterns, temperature, precipitation, winds and their extremes, in: Climate of the Mediterranean Region-From the Past to the Future, edited by: Lionello, P., Elsevier, 301–346, https://doi.org/10.1016/B978-0-12-416042-2.00005-7, 2012.
Unal, Y. S., Tan, E., and Mentes, S. S.: Summer heat waves over western Turkey between 1965 and 2006, Theor. Appl. Climatol., 112, 339–350, https://doi.org/10.1007/s00704-012-0704-0, 2013.
United Nations, Department of Economic and Social Affairs, Population Division: World Urbanization Prospects: The 2018 Revision (ST/ESA/SER.A/420), New York, United Nations, https://population.un.org/wup/Publications/Files/WUP2018-Report.pdf (last access: 5 April 2022), 2019.
Vahedifard, F., AghaKouchak, A., and Jafari, N. H.: Compound hazards yield Louisiana flood, Science, 353, 1374, https://doi.org/10.1126/science.aai8579, 2016.
Varlas, G., Anagnostou, M. N., Spyrou, C., Papadopoulos, A., Kalogiros, J., Mentzafou, A., Michaelides, S., Baltas, E., Karymbalis, E., and Katsafados, P. A.: Multi-Platform Hydrometeorological Analysis of the Flash Flood Event of 15 November 2017 in Attica, Greece, Remote Sens., 11, 45, https://doi.org/10.3390/rs11010045, 2019.
Vicente-Serrano, S. M., López-Moreno, J. I., Lorenzo-Lacruz, J., El Kenawy, A., Azorin-Molina, C., Morán-Tejeda, E., Pasho, E., Zabalza, J., Beguería S., and Angulo-Martínez, M.: The NAO impact on droughts in the Mediterranean region, in: Hydrological, socioeconomic and ecological impacts of the north Atlantic oscillation in the mediterranean region, Springer, Dordrecht, 23–40, 2011.
Vogel, J., Paton, E., Aich, V., and Bronstert, A.: Increasing compound warm spells and droughts in the Mediterranean Basin, Weather Climate Extremes, 32, 100312, https://doi.org/10.1016/j.wace.2021.100312, 2021.
Waha, K., Krummenauer, L., Adams, S., Aich, V., Baarsch, F., Coumou, D., Fader, M., Hoff, H., Jobbins, G., Marcus, R., Mengel, M., Otto, I. M., Perrette, M., Rocha, M., Robinson, A., and Schleussner, C. F.: Climate change impacts in the Middle East and Northern Africa (MENA) region and their implications for vulnerable population groups, Reg. Environ. Chang., 17, 1623–1638, https://doi.org/10.1007/s10113-017-1144-2, 2017.
Wilhite, D. A. and Glantz, M. H.: Understanding: The Drought Phenomenon: The Role of Definitions, Water Int., 10, 111–120, https://doi.org/10.1080/02508068508686328, 1985.
Williams, C. A.: Heat and drought extremes likely to stress ecosystem productivity equally or more in a warmer, CO2 rich future, Environ. Res. Lett., 9, 101002, https://doi.org/10.1088/1748-9326/9/10/101002, 2014.
World Meteorological Organization (WMO) and Global Water Partnership (GWP): Handbook of Drought Indicators and Indices, edited by: Svoboda, M. and Fuchs, B. A., Integrated Drought Management Programme (IDMP), Integrated Drought Management Tools and Guidelines Series 2, Geneva, ISBN 978-92-63-11173-9, 2016.
Yosef, Y., Saaroni, H., and Alpert, P.: Trends in daily rainfall intensity over Israel 1950/1-2003-4, Open Atmospheric Science Journal, 3, 196–203, 2009.
Yosef, Y., Aguilar, E., and Alpert, P.: Changes in extreme temperature and precipitation indices: using an innovative daily homogenized database in Israel, Int. J. Climatol., 39, 5022–5045, https://doi.org/10.1002/joc.6125, 2019.
Yosef, Y., Aguilar, E., and Alpert, P.: Is it possible to fit extreme climate change indices together seamlessly in the era of accelerated warming?, Int. J. Climatol., 41, E952–E963, https://doi.org/10.1002/joc.6740, 2021.
Yucel, I. and Onen, A.: Evaluating a mesoscale atmosphere model and a satellite-based algorithm in estimating extreme rainfall events in northwestern Turkey, Nat. Hazards Earth Syst. Sci., 14, 611–624, https://doi.org/10.5194/nhess-14-611-2014, 2014.
Zappa, G., Hawcroft, M. K., Shaffrey, L., Black, E., and Brayshaw, D. J.: Extratropical cyclones and the projected decline of winter Mediterranean precipitation in the CMIP5 models, Clim. Dynaam., 45, 1727–1738, 2015a.
Zappa, G., Hoskins, B. J., and Shepherd, T. G.: The dependence of wintertime Mediterranean precipitation on the atmospheric circulation response to climate change, Environ. Res. Lett., 10, 104012, https://doi.org/10.1088/1748-9326/10/10/104012, 2015b.
Zhang, W., Villarini, G., Scoccimarro, E., and Napolitano, F.: Examining the precipitation associated with medicanes in the high-resolution ERA-5 reanalysis data, Int. J. Climatol., 41, E126–E132, https://doi.org/10.1002/joc.6669, 2021.
Zhang, X., Aguilar, E., Sensoy, S., Melkonyan, H., Tagiyeva, U., Ahmed, N., Kutalade, N., Rahimzadeh, F., Taghipour, A,. Hantosh, T. H., Alpert, P., Semawi, M., Ali, M. K., Al-Shabibi, M. H. S., Al-Oulan, Z., Zatari, T., Khelet, I. A. D., Hamoud, S., Sagir, R., Demircan, M., Eken, M., Adiguzel, M., Alexander, L., Peterson, T. C., and Wallis, T.: Trends in Middle East climate indices from 1950 to 2003, J. Geophys. Res.-Atmos, 110, D22104, https://doi.org/10.1029/2005JD006181, 2005.
Zhang, X., Alexander, L., Hegerl, G. C., Jones, P., Tank, A. K., Peterson, T. C., Trewin, B., and Zwiers, F. W.: Indices for monitoring changes in extremes based on daily temperature and precipitation data, Wiley Interdiscip. Rev., 2, 851–870, 2011.
Zittis, G., Hadjinicolaou, P., and Lelieveld, J.: Role of soil moisture in the amplification of climate warming in the Eastern Mediterranean and the Middle East, Clim. Res., 59, 27–37, https://doi.org/10.3354/cr01205, 2014.
Zittis, G., Hadjinicolaou, P., Fnais, M., and Lelieveld, J.: Projected changes in heat wave characteristics in the eastern Mediterranean and the Middle East, Reg. Environ. Change., 16, 1863–1876, 2016.
Zittis, G.: Observed rainfall trends and precipitation uncertainty in the vicinity of the Mediterranean, Middle East and North Africa, Theor. Appl. Climatol., 134, 1207–1230, https://doi.org/10.1007/s00704-017-2333-0, 2018.
Zittis, G., Hadjinicolaou, P., Klangidou, M., Proestos, Y., and Lelieveld, J.: A multi-model, multi-scenario, and multi-domain analysis of regional climate projections for the Mediterranean, Reg. Environ. Change, 19, 2621–2635, https://doi.org/10.1007/s10113-019-01565-w, 2019.
Zittis, G., Bruggeman, A., and Camera, C.: 21St Century Projections of Extreme Precipitation Indicators for Cyprus, Atmosphere-Basel, 11, 343, https://doi.org/10.3390/atmos11040343, 2020.
Zittis, G., Hadjinicolaou, P., Almazroui, M., Bucchignani, E., Driouech, F., El Rhaz, K., Kurnaz, L., Nikulin, G., Ntoumos, A., Ozturk, T., and Proestos, Y.: Business-as-usual will lead to super and ultra-extreme heatwaves in the Middle East and North Africa, npj Clim. Atmos. Sci., 4, 1–9, 2021a.
Zittis, G., Bruggeman, A., and Lelieveld, J.: Revisiting future extreme precipitation trends in the Mediterranean, Weather Clim. Extrem., 34, 100380, https://doi.org/10.1016/j.wace.2021.100380, 2021b.
Ziv, B. and Saaroni, H.: The contribution of moisture to heat stress in a period of global warming: the case of the Mediterranean, Climatic Change, 104, 305–315, https://doi.org/10.1007/s10584-009-9710-3, 2011.
Ziv, B., Saaroni, H., Yair, Y., Ganot, M., Baharad, A., and Isaschari, D.: Atmospheric factors governing winter thunderstorms in the coastal region of the eastern Mediterranean, Theor. Appl. Climatol., 95, 301–310, 2009.
Ziv, B., Saaroni, H., Pargament, R., Harpaz, T., and Alpert, P.: Trends in rainfall regime over Israel, 1975-2010, and their relationship to large-scale variability, Reg. Environ. Change, 14, 1751–1764, https://doi.org/10.1007/s10113-013-0414-x, 2014.
Ziv, B., Harpaz, T., Saaroni, H., and Blender, R.: A new methodology for identifying daughter cyclogenesis: application for the Mediterranean basin, Int. J. Climatol., 35, 3847–3861, https://doi.org/10.1002/joc.4250, 2015.
Zoccatelli, D., Marra, F., Armon, M., Rinat, Y., Smith, J. A., and Morin, E.: Contrasting rainfall-runoff characteristics of floods in desert and Mediterranean basins, Hydrol. Earth Syst. Sci., 23, 2665–2678, https://doi.org/10.5194/hess-23-2665-2019, 2019.
Zoccatelli, D., Marra, F., Smith, J. A., Goodrich, D., Unkrich, C. L., Rosensaft, M., and Morin, E.: Hydrological modelling in desert areas of the eastern Mediterranean, J. Hydrol., 587, 124879, https://doi.org/10.1016/j.jhydrol.2020.124879, 2020.
Zscheischler, J. and Seneviratne, S. I.: Dependence of drivers affects risks associated with compound events, Science Advances, 3, e1700263, https://doi.org/10.1126/sciadv.1700263, 2017.
- Article
(10388 KB) - Full-text XML