the Creative Commons Attribution 4.0 License.
the Creative Commons Attribution 4.0 License.
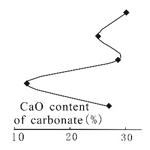
Characteristics of soil profile CO2 concentrations in karst areas and their significance for global carbon cycles and climate change
Qiao Chen
CO2 concentrations of 21 soil profiles were measured in Zhaotong City, Yunnan Province. The varying characteristics of soil profile CO2 concentrations are distinguishable between carbonate and noncarbonate areas. In noncarbonate areas, soil profile CO2 concentrations increase and show significant positive correlations with soil depth. In carbonate areas, however, deep-soil CO2 concentrations decrease and have no significant correlations with soil depth. Soil organic carbon is negatively correlated with soil CO2 concentrations in noncarbonate areas. In carbonate areas, such relationships are not clear. This means that the special geological process in carbonate areas – carbonate corrosion – absorbs part of the deep-soil-profile CO2. Isotope and soil pH data also support such a process.
A mathematical model simulating soil profile CO2 concentration was proposed. In noncarbonate areas, the measured and the simulated values are almost equal, while the measured CO2 concentrations of deep soils are less than the simulated in carbonate areas. Such results also indicate the occurrence of carbonate corrosion and the consuming of deep-soil CO2 in carbonate areas. The decreased CO2 concentration was roughly evaluated based on stratigraphic unit and farming activities. Soil pH and the purity of CaCO3 in carbonate bedrock deeply affect the corrosion. The corrosion in carbonate areas decreases deep-soil CO2 greatly (accounting for 5.2 %–66.3 % with average of 36 %) and naturally affects the soil CO2 released into the atmosphere. Knowledge of this process is important for karst carbon cycles and global climate changes and it may be a part of the “missing carbon sink”.
In recent years, there has been increasing worldwide concern about carbon exchange among the atmosphere, the ocean, and terrestrial ecosystems. Specifically, there have been ongoing questions regarding the problem of carbon flux, or carbon source versus carbon sink. The missing carbon sink has puzzled scientists since Callendar (1938) presented the imbalance of absorbed and released CO2. The missing carbon sink reaches as much as 1.7 Pg, accounting for as much as 24 % of total carbon (Sundquist, 1993). There are differing viewpoints regarding the spatial distribution and absorption strength of the missing carbon sink in terrestrial ecosystems (Fan et al., 1998; Potter and Klooster, 1999). The carbon cycle in karst areas has attracted great interest due to the absorbed and released CO2 via carbonate corrosion and its share in regulating atmospheric CO2 (Li and Yuan, 1995; Martin et al., 2013). Therefore, some scholars have looked for the “missing sink” within the absorbed and released carbon in karst systems, and the estimated values reach a dominating part (almost one-third) of the missing sink (Jiang and Yuan, 1999).
Soil carbon, with storage of 1300–2000 Pg C, and as much as 2–3 times that of vegetation storage, plays an important role in maintaining carbon balance (Fearnside, 2018) so that a slight change imposes a great effect on the atmospheric CO2 concentration. Several factors affecting soil CO2 concentration, such as environmental factors (soil temperature, moisture, water content, etc.) and human activities, have been widely discussed (Bajracharya et al., 2000; Dai et al., 2004; Owens et al., 2018; Fearnside, 2018). In karst areas, however, the important geological process, carbonate corrosion, has been largely ignored in discussions of soil CO2 levels and there is no documents detailing the soil CO2 concentration and its relationship with global climate change in karst areas. Several problems puzzle us. Is there any difference between soil profile CO2 concentrations in carbonate areas and those in noncarbonate areas? If so, is the difference caused by carbonate corrosion? By how much is it affected? Moreover, studies have revealed that there is a CO2 imbalance between carbon released into atmosphere and that produced by organic matter in carbonate areas (Jiang and Yuan et al., 1999; Pan et al., 2000) but there is no reasonable explanation. Lack of research work on these questions restricts our understanding of soil CO2 transfer, limits further study of the mechanisms, and impedes learning of its significance for the carbon cycle.
In order to understand the varying characteristics of soil CO2 concentrations in karst areas and their potential effect on the global carbon cycle, soil profile CO2 was measured and samples of soils and rocks were gathered in the typical karst area of Zhaotong City, Yunan Province, China. The objectives of this paper are to (1) analyze comparatively the varying characteristics of the soil profile CO2 concentration in carbonate and noncarbonate areas; (2) discuss the relationship between soil CO2 concentration and other parameters, and clarify the effect of carbonate corrosion on soil CO2; and (3) develop a mathematical model of soil CO2 transfer and quantitatively evaluate the effect scale of carbonate corrosion on soil CO2 concentration, and discuss its significance for the global carbon cycle and climate change.
2.1 Study area
The study area including Zhenxiong County and Weixin County in Zhaotong City, northern Yunnan Province, China, was selected. The area contains high mountains and steep gorges. Many of the mountain peaks tower above 2000 m and there are many different natural watersheds. The area is subtropical and humid. It has a plateau climate with an average annual temperature of 11.7 ∘C and an average precipitation of 1200 mm. Monthly precipitation is above 100 mm and vertical climate belts with four seasons are clearly demarcated. The soil types include mainly yellow, black and brown earth with a wide thickness range (from a few centimeters up to 70–80 cm). The flora is dominated by grass, shrubs, and partly by secondary forest.
The bedrock is composed predominantly of Mesozoic limestone and dolomite with flysch and associated sedimentary rocks. The widely exposed strata include mainly Ordovician, Permian, Triassic, Jurassic, and Quaternary units. Devonian strata are not present and Precambrian, Cambrian, and Silurian strata occur in limited outcrops or as inclusions among other strata. Ordovician, Permian, and Triassic rocks are mainly marine carbonate deposits and Jurassic and Quaternary units are mainly composed of terrestrial clastic deposits.
2.2 Sampling and analyzing methods
In order to comprehensively reveal characteristics of soil CO2 concentration in the karst area, soil profiles of different stratigraphic units and vegetation types were selected. And profiles in carbonate or noncarbonate areas were both involved. In total, CO2 concentrations of 21 soil profiles and organic carbon of 12 soil profiles were analyzed. The profile sites are shown in Fig. 1, and among these profiles in carbonate areas include the Lower Ordovician Meitan Formation (O1), the Middle and Upper Ordovician Baota Formation (O2−3), the Lower Permian Xixia and Maokou formations (P1m(q)), the Upper Permian Changxing Formation (P2c), and the Middle Triassic Guanling Formation (T2g). Sites in noncarbonate areas include Middle Permian basalt (P2β), shale in the Upper Permian Longtan Formation (P2l), mudstone in the Lower Triassic Feixianguan Formation (T1f), and siltstone intercalated with shale in the Upper Triassic Xujiahe Formation (T3x).
CO2 concentration within the soil pores was measured every 10 cm from the surface down to the rock–soil interface using a GASTEC 801 instrument and 2LL or 2L CO2 detector tube (GASTEC Co., Japan). The profile soil samples were of one-to-one correspondence with the gas samples and also taken every 10 cm.
The starting samples were air-dried naturally and then pulverized (particle diameter < 150 µm). Soil organic carbon was determined using the potassium dichromate volumetric method. Soil pH was measured in distilled water at a solid/solution ratio of 1∕5, with the instrument model PHS-2. Water contents of soils were synchronously measured by a cutting ring. CaO and MgO contents of rocks were determined by inductively coupled plasma atomic emission spectrometry (ICP-AES) with a charge injection detector (CID), model TJA IRIS/AP. The standard materials (GBW07401, GBW07408) were used for quality control with relative deviation less than 5 %.
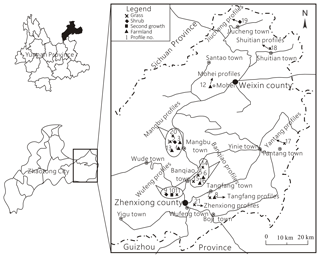
Figure 1Sites for measuring soil CO2 and gathering organic carbon samples (1 is Mangbu O2−3 grass, 2 is Mangbu O2−3 shrub, 3 is Mangbu O2−3 farmland, 4 is Mangbu O2−3 farmland, 5 is Banqiao O1m grass, 6 is Mangbu O1m farmland, 7 is Tangfang P2c grass, 8 is Tangfang P2c farmland, 9 is Wufeng P2l shrub, 10 is Wufeng P2l second growth, 11 is Wufeng P2l grass, 12 is Mohei P2l farmland, 13 is Mangbu O2−3 farmland, 14 is Banqiao P2c grass, 15 is Banqiao P1m(q) grass, 16 is Banqiao P1m(q) shrub, 17 is Tangfang P2β grass, 18 is Shuitian T2g shrub, 19 is Jiucheng T3x shrub, 20 is Mangbu O1m grass, and 21 is Zhenxiong T1f grass).
3.1 Varying CO2 concentration characteristics of soil profiles
Figure 2 shows soil profile CO2 concentrations varying with soil depths in seven noncarbonate areas. The data show a distinct tendency of increasing CO2 concentration with soil depth with R2=0.8–0.92 (Table 1). The reasons may be the higher soil bulk density, more condensed soil pores, and difficulty of CO2 diffusion in the deeper soil. In fact, soil profile CO2 has been widely reported to be correlated with soil depth by previous research (Rustad et al., 2000; Dai et al., 2004; Malak et al., 2018) and even the following linear equation has been developed (James and George, 1991). Mean CO2 ). Our observations in noncarbonate areas are concordant with these reports and support soil profile CO2 increases with soil depth in noncarbonate areas.
Table 1Regression analysis of soil CO2 concentration and profile depth in noncarbonate areas.
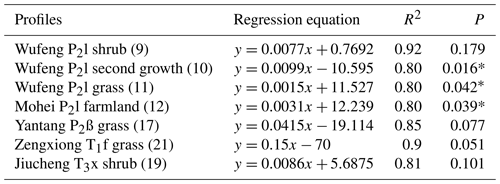
Note: numbers in parenthesis give the profile no., ∗ means significant correlation at 0.05 level.
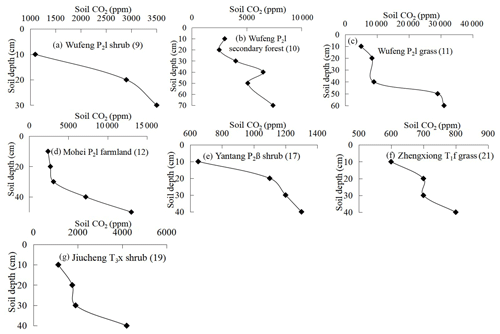
Figure 2Varying characteristics of soil profile CO2 concentration in noncarbonate areas (profile no. in brackets).
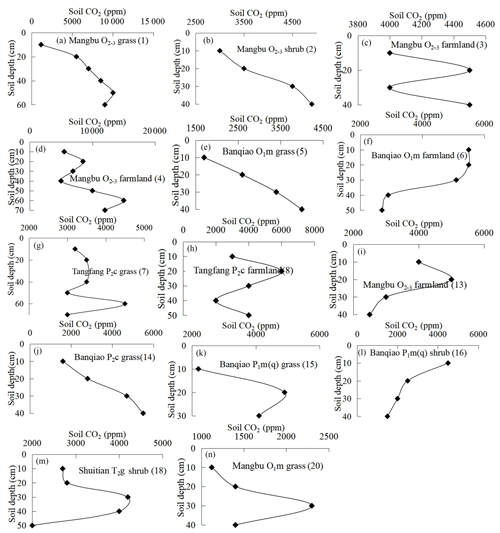
Figure 3Varying characteristics of soil profile CO2 concentration in carbonate areas (profile no. in brackets).
Fourteen soil profile CO2 concentrations with soil depth in carbonate areas were gained (Fig. 3). The results show a complex and inverse relationship between soil CO2 and soil depth in carbonate areas. Most soil profile CO2 increases with soil depth in the upper sections, such as the Mangbu O2−3 grassy profile (Fig. 3a), Mangbu O2−3 shrub profile (Fig. 3b), Mangbu O2−3 farmland profile (Fig. 3d), Banqiao O1m grassy profile (Fig. 3e), and Banqiao P2c grassy profile (Fig. 3j). CO2 concentrations decrease with soil depth when they increase from surface to a certain depth in Mangbu O2−3 farmland profile (Fig. 3i), Banqiao P1m(q) grassy profile (Fig. 3k), Gaotian T2g grassy profile (Fig. 3m), and Mangbu O1m grassy profile (Fig 3n). Those of Banqiao O1m farmland profile (Fig. 3f) and Banqiao P1m(q) shrub profile (Fig. 3l) even decrease all along with soil depth; two farmland profiles of Mangbu O2−3 (Fig. 3c) and Tangfang P2c (Fig. 3h) fluctuate and have no regularity due to the effect of human farming activities. Generally, except Mangbu O2−3 farmland profile (Fig. 3c) and Tangfang P2c farmland profile (Fig. 3h), which are disturbed by farming, CO2 concentrations of other profiles in carbonate areas all decrease with soil depth at the rock–soil interface (Fig. 3b, e, j). Moreover, there is no correlation of soil CO2 concentration with soil depth because sequestration of deep-soil CO2 concentration occurs in carbonate areas. Why does the sequestration only take place in carbonate areas but not in noncarbonate ones? Naturally, the particular carbonate process – carbonate corrosion – is considered, i.e., part of deep-soil CO2 is consumed and CO2 sequestration occurs and there is no linear relationship between CO2 concentration and soil depth in carbonate areas. In fact, Buyannovsky and Wagner (1983), Solomon and Cerling (1987), and Xu and He (1996) all reported that soil CO2 concentrations reach a peak at a certain depth and then decrease with soil depth in carbonate areas. CO2 concentrations in Banqiao O1m farmland profile (Fig. 3f) and Banqiao P1m(q) shrub profile (Fig.3l) continue to decrease with depth through the integral profile and they also had the highest concentration at the 10 cm layer. Instances of CO2 concentrations in surface layers higher than those in bottom layers are scarcely documented in carbonate areas.
3.2 Relationship between soil profile CO2 concentrations and soil organic carbon
Soil organic carbon (SOC) was analyzed in a part of the profiles, corresponding with CO2 concentration. Results are given in Fig. 4, among which panels 4a–h indicate profiles in carbonate areas and panels 4i–l indicate those in noncarbonate (shale) areas.
Correlation analysis of soil profile CO2 concentration and SOC in shale areas is listed in Table 2. CO2 shows a decreasing tendency with increasing SOC with high regression coefficients (R2=0.67–0.85). An exception of 0.29 occurs in Wufeng P2l secondary forest, which possibly is caused by stronger root respiration and a higher ratio of CO2 generated by the roots. Therefore, SOC is directly affected by the release of soil CO2 and the key problem for soil carbon storage is to slow down the renewing of soil organic matter (Chen et al., 2002). The reason for nonsignificance (P>0.05) may be that soil CO2 concentration is related not only to SOC but also to soil respiration and microbe activities. However, there is no such tendency in carbonate areas as that in shale areas (Table 3) and even those of Banqiao O1m farmland profile and Banqiao P1m(q) shrub profile show an increasing tendency. Previous studies in carbonate areas such as Shilin, Lunan City, and Guizhou Plateau also showed no correlation between CO2 concentration and SOC (Liang et al., 2003).
What is the reason for the poor relationship between soil CO2 and SOC in carbonate areas? A possible answer may be carbonate corrosion. By means of corrosion, deep-soil CO2 is partly consumed and its level decreases. Consequently, the relationship becomes poor. In addition, varying characteristics of SOC cannot explain well the decrease in deep-soil CO2 levels in carbonate areas.
3.3 Varying characteristics of profile soil pH
Soil pH curves varying with soil depth are drawn in Fig. 5a–h, indicating carbonate profiles, and Fig. 6i–l, indicating noncarbonate (shale) profiles. In noncarbonate areas, there is a complex relationship between pH and depth; pH increases obviously at the rock–soil interface, whereas pH nonsignificantly varied with soil CO2 and SOC. Conversely, in carbonate areas, pH generally increases with soil depth in the surface layer except in the Banqiao O1m farmland profile. Moreover, from Figs. 3 and 5 it is evident that soil CO2 concentrations decrease where soil pH decreases too, and even CO2 levels in the Banqiao O1m farmland profile decrease from the surface to the bottom with soil pH through the entire profile. These observations imply that the decrease in deep-soil CO2 concentration in carbonate areas is related closely to soil pH.
Chemically, with soil water and soil CO2 added together, carbonate corrosion can be represented by the following reaction:
By means of this reaction, deep-soil CO2 is consumed by the corrosion of the underlying carbonate rock and pH decreases synchronously. This reaction cannot take place in soil over areas with noncarbonate bedrock so here the deep-soil CO2 concentration does not decrease, but increases.
3.4 Carbonate corrosion and the global carbon cycle
Many studies have observed that soil CO2 concentration in carbonate areas decreases with depth when it reaches a maximum at a certain soil depth in carbonate areas (Buyannovsky and Wagner, 1983; Li and Yuan, 1995; Xu and He, 1996; Liang et al., 2003). There has, however, been no reasonable explanation for the observations. Li and Yuan (1995) attributed it to less roots, and therefore less root respiration in the deep soil, but there are no scientifically observed data to support this idea and it remains only a hypothesis. No decrease in soil CO2 in noncarbonate areas is found, and, furthermore, the depths with decreasing CO2 concentrations were distinguishable in different profiles, even at only 20–30 cm depths. The decreased CO2 concentration could be attributed to decreased microbe numbers or root respiration at such depths. By comparative analysis of soil CO2 concentration in areas of carbonate and noncarbonate bedrock, it should be suggested that the explanation is due to the special geological process of carbonate corrosion.
Soil CO2 and SOC in noncarbonate areas have a good negative correlation with correlation coefficients R2=0.67 to 0.85, although significance is not clear because soil CO2 is determined not only by organic matter but also by other factors such as root respiration and microbe activities. By contrast, such a correlation in carbonate areas is poor, which was concluded also by Li and Yuan (1995) and Liang et al. (2003) from experiments in carbonate areas. Soil CO2 of carbonate areas, in every depth at different sites, is negatively correlated with SOC and the relationship becomes worse with increasing soil depth. This observation means that SOC content cannot explain well the decreased CO2 concentration of deep soil in carbonate areas but rather may be related to carbonate corrosion. Soil pH in carbonate areas always decreases with soil CO2 and this may imply that H+ generated by carbonate corrosion mixes into the deep soil increasing soil acidity.
Previous work has determined the imbalance between soil CO2 produced and released in carbonate areas. Pan et al. (2000) observed and simulated field data in Yaji, Guangxi Province, concluding that CO2 produced by decomposition of organic matter is more than that released into the air. This confirms that the rock and the soil have an obviously “absorbing effect” for CO2. The data account for an absorbing coefficient of 22–130 g m−2 a−1.
Isotopes can effectively trace the carbon source of soil CO2. Figure 6 reflects the δ13C value of soil CO2 and SOC overlying different bedrock types according to data from Li et al. (2001). It shows that in deep soil, CO2 has a higher δ13C value than the SOC in limestone and dolomite areas, whereas the isotope ratios are more equivalent in clay-stone areas. Such an observation may support the conclusion that deep-soil CO2 in clay-stone areas is mainly or completely from soil organic matter and that in limestone and dolomite areas there must be an additional carbon source whose δ13C should be more than −14 ‰ . CaCO3 in carbonate has δ13C values of −3 ‰ to ‰. It must, therefore, be recognized that carbon in CaCO3 of carbonate bedrock mixes into soil CO2 since the corrosion reaction is reversible.
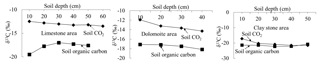
Figure 6Varying δ13C of soil CO2 and soil organic carbon with soil depth overlying different bedrock types (data are after Li et al., 2001).
It has been examined that the karst carbon cycle is an important trace for the global carbon cycle and that further study is important to the hunt for the missing carbon sink (Jiang and Yuan, 1999). From what is presented above, with a focus on the process of carbonate corrosion and comparison of different parameters in carbonate and noncarbonate areas, it is logical to conclude that carbonate corrosion causes the decreased CO2 concentration at the rock–soil interface in carbonate areas. As a result, the decreased CO2 level caused by corrosion will, of course, impose effects on atmospheric CO2 and the karst carbon cycle. This is significant for the potential fixation of carbon, the study of global carbon cycle balance, and the hunt for the missing carbon sink.
3.5 Mathematical model of soil profile CO2 transfer
In this model, only the molecular diffusion of CO2 is considered, neglecting other processes such as viscous flow and Knudsen diffusion in karst soil because of the weak air pressure gradient. Moreover, density gradient was regarded as the dominant dynamic of CO2 diffusion and temperature gradient was neglected because of its low contribution (0.2 %–0.4 %) to CO2 flow. Therefore, the transport of soil CO2 can be described by the following one-dimensional diffusion equation according to Fick's second law and laws of conservation of mass (Zeng and Zheng, 2002), assuming horizontal homogeneity:
Here, θa is the air content, θw is the water content, Ca is the gaseous CO2 concentration, Jda is the gaseous CO2 flow due to diffusion, Jdw is the dissolution CO2 flow due to diffusion, Jca is the gaseous CO2 flow due to convection, Jcw is the dissolution CO2 flow due to convection, S is the carbon source, Q is the water absorbed by roots, t is time, and z is the space coordinate.
Such an equation can be gained according to Fick's first law:
where Da is the gaseous CO2 diffusion coefficient in soil substrate, Dw is the dissolution CO2 diffusion coefficient in soil substrate, qa is the soil air transference amount, and qw is the soil water transference amount.
Equation (3) can be deduced from Eqs. (1) and (2) if it is assumed that soil water is stable and gaseous and dissolution CO2 flows are not considered:
Previous studies were referenced when the parameters were determined and all the parameters should be gained in winter of the same working period: , presented by Yoyam and Dani (1993), and by Warren and Michael (1984), and in winter qw=0 and Q=0; , by Collin and Rasmuson (1988). Here, is the CO2 diffusion coefficient in air at the reference temperature T0.
For the carbon source, the rate of CO2 produced by root respiration and microbes can be expressed as follows: , where S(z) is the soil profile CO2 at depth of z, S0 is the CO2 concentration in the surface soil, z is the soil depth, and zs is the depth gradient. It also considered the CO2 produced by organic matter expressed as follows: .
Then Eq. (4) is achieved:
where and are stable when they are from the same time and soil profile.
Based on the studies above, the soil profile CO2 concentration varying with soil depth can be expressed by the following equation:
According to Tailor's formula
and it can be roughly expressed like the following equation when x < 1:
When Eq. (7) is applied to Eq. (5), Eq. (8) can be gained to express profile CO2 concentration (Ca) varying with soil depth (z):
Here, a, b, and c are uncertain parameters, which vary with θa, θw, S0, T, and Da of different profiles.
That means it can be expressed as a linear or parabolic relationship of soil profile CO2 concentration and soil depth. Actually, many observations and simulations also confirmed the same results (James and George, 1991; Zeng and Zheng, 2002; Malak et al., 2018). Therefore, it seems reasonable to express a linear or parabolic relationship of soil profile CO2 concentration and soil depth.
3.6 The rough evaluation of CO2 decreased by corrosion
SPSS software was used to simulate the curve of measured soil CO2 concentration and soil depth in noncarbonate areas (Fig. 7 and Table 4), resulting in parabolas with multiple regression coefficients R2=0.8 to 1. Multiple regression coefficient of P2c secondary forest profile shows the lowest level at 0.79, which may be due to the different root respiration and the absorbed water at different depths. The simulation evidence that the model is reliable and can be used to roughly reveal the laws of soil profile CO2 concentration.
Table 4Simulated equation of measured soil CO2 concentration and soil depth in noncarbonate areas.
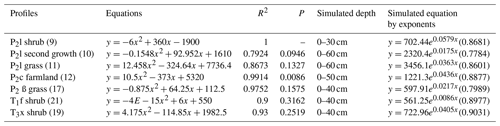
Note: regression coefficients R2 of simulated exponent in brackets.
In carbonate areas, however, there is no linear or parabolic relationship between soil profile CO2 concentration and soil depth and the measured values are inconsistent with the simulated ones. Linear or parabolic relationships can be found in the surface soil. Since it is carbonate corrosion that decreases the CO2 concentration in the deep soil of carbonate areas, the CO2 concentration in the surface layer can be used to predict the CO2 concentration of deep soil based on the developed model. The predicting equation and results are listed in Fig. 8. It shows that there is a strong difference between the measured and the predicted values and that all the predicted values are greater than the measured ones in deep soil. It can also be deduced that deep-soil CO2 is consumed by carbonate corrosion.
The method of subtraction of predicted and measured values can be used to evaluate the decreased CO2 concentration in carbonate areas caused by carbonate corrosion, and the results are listed in Table 5. If synthesis factors, such as vegetation types and soil types, were considered, the rough evaluation of the decreased CO2 concentration of every stratigraphic unit can be gained by taking the average (Fig. 9).
Table 5The evaluated results of the decreased CO2 concentration in carbonate areas caused by carbonate corrosion.

3.7 The main controlling factors of decreased CO2 concentration
Figure 9 shows great dissimilarity in the decreased CO2 concentration with different stratigraphic units in the following order: T2g > P1m(q) > O1m > O2−3 > P2c. Figure 10 shows the calculated results of the decreased CO2 concentration in farmland and natural soil (grass and shrub, respectively) of the same stratigraphic unit. CO2 concentration in T2g and P1m(q) farmland is lacking but the comparative analysis of O1m , O2−3, and P2c can demonstrate that the decrease in CO2 in natural soil profiles is obviously less than that in farmland profiles. It is clear that corrosion was strengthened by farming activities and more CO2 was consumed in the deep soil, which may be due to higher CO2 levels and acidity caused by farming. Therefore, the decreased CO2 concentrations of T2g and P1m(q) should be more than the calculated values, when farming activities are considered. The decreased CO2 concentration in different farmland profiles is remarkably distinguishable at different sites, even on their same stratigraphic units (Table 5). It seems that the degree of human activity and the quantities of imported or exported energy determine the corrosion to some degree.
Several parameters, such as CaO and MgO contents of carbonate, water content, and pH of the overlying soil, were determined to address some natural factors affecting decreased CO2 concentration. The parameters are shown in Fig. 11. Deep-soil pH is negatively correlated with decreased CO2 concentration and the stronger the soil acidity, the more the decreased CO2 concentration. Water content of deep soil does not impose an effect on corrosion. CaO content of carbonate is positively correlated with the decreased CO2 concentration and the purer the CaCO3 in carbonate rock, the stronger is the corrosion. MgO content of carbonate is not correlated with corrosion, which indicates that it is CaCO3 corrosion and not that of MgCO3 that is consuming soil CO2. Simulation by SPSS software results in an equation () of decreased CO2 concentration and soil pH with a multiple regression coefficient R2=0.9779 and a second equation () of decreased CO2 level and CaO content of carbonate with a multiple regression coefficient R2=0.4191 (Fig. 12). A field experiment of carbonate corrosion in the southern part of Guizhou (Nie et al., 1984), a laboratory simulation using citric acid to corrode limestone (Cao et al., 2001), and an experimental study on the stability of CaCO3 and MgCO3 under acid rain conditions (Teir et al., 2006) led to the conclusion that corrosion is related closely with soil acidity and carbonate purity. The calculated results can support the same conclusion and accord well with their studies.
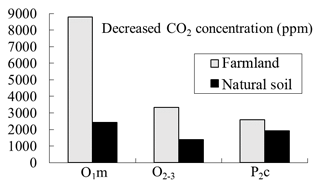
Figure 10The decreased CO2 concentration in farmland and natural soil of the same stratigraphic unit.
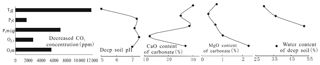
Figure 11Relationship of the decreased CO2 concentration and deep-soil pH, water content, CaO, and MgO contents of carbonate.
It is not surprising that soil CO2 concentrations decrease in the deep layers over carbonate bedrock areas, especially at the bottom of soil profiles, as has been observed by many experiments (Buyannovsky and Wagner, 1983; Li and Yuan, 1995; Xu and He, 1996; Liang et al., 2003), and is now supported by this paper. The explanation by some studies (Li and Yuan, 1995) that decreased CO2 is caused by decreased microbe or root respiration in deep soil is challenged by our data. At first, one important reason leading to the earlier conclusion lies, perhaps, in the lack of comparative analyses of soil CO2 levels in carbonate and noncarbonate areas. The underlying foundation of soluble carbonate in carbonate areas was not taken into consideration, and, most importantly, there was no proof or data to support this idea. Secondly, there is no decrease in CO2 in soil profiles of noncarbonate areas (mudstone, basalt, shale, or siltstone areas); also, it seems reasonable to expect CO2 decrease by lower microbe and root respiration rates in deep soil layers of both carbonate or noncarbonate areas. Thirdly, decrease in soil CO2 takes place in 20–30 cm soil layers, and even from the soil surface in some profiles, so it may be unreasonable to attribute CO2 decrease to microbe respiration in such shallow occurrences.
Additionally, soil profile CO2 only decreases in carbonate areas and SOC content is positively correlated with soil CO2 concentration in noncarbonate areas (R2=0.67–0.85), although there is no significant correlation in some profiles because soil CO2 is not only related with organic carbon but also with other factors such as root respiration. Soil CO2 and organic carbon in different depths of carbonate areas are positively correlated with low correlation coefficients but not in soil profiles of these carbonate areas. This means that organic carbon cannot be responsible for the decreased CO2 concentrations. Furthermore, CO2 consumed by carbonate corrosion leads to an uncorrelated relationship between soil CO2 and organic carbon levels in carbonate areas. Soil profile pH in carbonate areas always suddenly and sharply decreases at the depth of CO2 decrease and this can be explained well by carbonate corrosion. Analysis of δ13C isotope, which mixes into a CO2 in deep soil layers of carbonate bedrock areas (dolomite or limestone) also demonstrates that there is another carbon source, whose δ13C level is more than −14 ‰. In soil of clay-stone areas, however, soil CO2 and soil organic carbon have the same δ13C value. This provides strong evidence that carbonate corrosion occurs, and thus deep-soil CO2 is consumed in carbonate areas. Simply stated, our work strongly indicates that carbonate corrosion leads to the decrease in soil profile CO2 concentration in areas with carbonate bedrock.
Further, a mathematical model of soil CO2 transfer was developed, showing that soil CO2 concentration can be roughly expressed as a linear or parabolic increase with soil depth. The linear or parabolic increase can be demonstrated, strongly supported by both field data and the models. Soil CO2 concentration data, collected in noncarbonate areas or in the surface soil of carbonate areas, provide additional confirmation. In the deep soil of carbonate areas, however, especially at the rock–soil interface, the simulated values are always higher than the field measurements. All of these points may also indicate that carbonate corrosion occurs in the deep soil and that a part of soil CO2 is consumed by carbonate corrosion. In addition, the decreased CO2 concentration caused by carbonate corrosion can be evaluated by the subtraction of measured and simulated CO2. The decreased CO2 concentration is related closely to deep-soil pH and CaO content of carbonate rock (correlation coefficients, respectively, R2=0.97 and 0.41), together with farming activities, but not with deep-soil water content and MgO content of carbonate. These results and conclusions can be supported by experiments and are widely accepted by karst scholars, who add validity to our results and conclusions.
The carbon cycle in karst areas has attracted great attention because of the imbalance of the global carbon cycle and in recent years there has been a search to resolve the missing carbon sink related to the absorbing and releasing of carbon in CaCO3 systems (Jiang and Yuan, 1999). Experiments and calculations indicate that 1.774×107 t of carbon are absorbed by karstification in China and that t of carbon are drawn back from the atmosphere worldwide every year (Jiang and Yuan, 1999). It is obviously significant in regards to the increasing atmospheric temperature. Soil, as an important carbon storage area, is of great importance to atmospheric CO2 concentration and slight variations may impose great effects on the global carbon cycle. Several factors affecting soil CO2 concentration have been discussed, such as environmental ones (soil temperature, moisture, water content, etc.), microbe activities, and human activities, but no published details about the effect of carbonate corrosion on soil CO2 concentration can be found. Our study argues that deep-soil CO2 concentrations in carbonate areas are obviously decreased, especially at the rock–soil interface, and that this is mainly caused by carbonate corrosion. If this conclusion is correct, then naturally the atmospheric CO2 levels in carbonate areas should be affected by the corrosion and this should be very significant in the hunt for the missing carbon sink.
The data used are available upon request from the corresponding author.
The author declares that there is no conflict of interest.
The author expresses heartfelt thanks to the staff of the Zhaotong Bureau of Sciences and Technology and the Institute of Geographic Sciences and Natural Resources Research (IGSNRR) for gathering the samples, as well as Craig Breckinridge Wood, Department of Biology Faculty, Providence College, USA, for correcting English grammar.
This research has been supported by the Natural Science Fund of Shandong Province (grant no. ZR2018MD012), the Chinese National Key Natural Science Foundation Grant Agreement (grant no. 90202017), and the 2017 Special Fund for Scientific Research of Shandong Coalfield Geologic Bureau (grant no. 2017(10)).
This paper was edited by Sagnik Dey and reviewed by two anonymous referees.
Bajracharya, R. M., Lai, R., and Kimble, J. M.: Erosion effects on carbon dioxide concentration and carbon flux from an Chio Alfisol, Soil Sci. Soc. Am. J., 64, 694–700, 2000.
Buyannovsky, G. A. and Wagner, G. H.: Annual cycles of carbon dioxide level in soil air, Soil Sci. Soc. Am. J., 47, 1139–1145, 1983.
Callendar, G. S.: The artificial production of carbon dioxide and its influence on temperature, Q. J. Roy. Meteor. Soc., 64, 223–240, 1938.
Cao, J. H., Pan, G. X., and Yuan, D. X.: Simulation experiments on dissolution of calcite by citric and its karst importance, Carsologica Sinica, 20, 1–4, 2001 (in Chinese).
Chen, Q. Q., Sun, Y. M., Shen, C. D., Peng, S. L., Yi, W. X., Jiang, M. T., and Li, Z. A.: Quantitative study on organic matter turnover characteristics of mountainous soil profiles in the subtropical area, south China, Scientia Geographical Sinica, 22, 196–201, 2002 (in Chinese).
Collin, M. and Rasmuson, A.: A comparison of gas diffusivity models for unsaturated porous media, Soil Sci. Soc. Am. J., 52, 1559–1565, 1988.
Dai, W. H., Wang, Y. Q., Huang, Y., Liu, J., and Zhao, L.: Seasonal dynamic of CO2 concentration in Lou soil and impact by environmental factors, Acta Pedologica Sinica, 41, 827–831, 2004 (in Chinese).
Fan, S., Gloor, M., Mahlman, J., Pacala, S., Sarmiento, J., Takahashi, T., and Tans, P.: A large terrestrial carbon sink in North American implied by atmospheric and oceanic carbon dioxide data and models, Science, 282, 442–446, 1998.
Fearnside, P. M.: Brazil's Amazonian forest carbon: the key to Southern Amazonian's significance for global climate, Reg. Environ. Change, 18, 47–61, 2018.
James, M. D. and George, A. B.: Spatial and temporal variations in temperate forest soil carbon dioxide during the non-growing season, Earth Surf. Proc. Land., 16, 411–426, 1991.
Jiang, Z. C. and Yuan, D. X.: Dynamics features of the epikarst zone and their significance in environments and resources, Acta Geoscientia Sinica, 20, 302–308, 1999 (in Chinese).
Li, B. and Yuan, D. X.: Carbon cycle of epikarst in carbonate areas, in: Earthquake Center, carbonate and environment (Vol. 1), edited by: Wan, G., Earthquake Press, Beijing, 1–15, 1995 (in Chinese).
Li, T. Y., Wang, S. J., and Zheng, L. P.: Comparative study on CO2 sources in soil developed on carbonate rock and non-carbonate rock in Central Guizhou, Sci. China Ser. D, 31, 777–782, 2001.
Liang, F. Y., Song, L. H., Tang, T., and Wang, J.: Microbial production of CO2 in red soil in stone forest national park, Carsologica Sinica, 22, 6–11, 2003 (in Chinese).
Malak, M. T., Nancy, J. H., Akihiro, K., and Evans, R. D.: Elevated CO2 changes soil organic matter composition and substrate diversity in an arid ecosystem, Geoderma, 330, 1–8, 2018.
Martin, J. B., Brown, A., and Ezell, J.: Do carbonate karst terrains affect the global carbon cycle?, Acta Carsologica, 42, 187–196, 2013.
Nie, Y. P.: A Preliminary study and test on the dissolution of carbonate rocks in south Guizhou province, Carsologica Sinica, 1, 39–45, 1984 (in Chinese).
Owens, J. D., Lyons, T. W., and Lowery, C. M.: Quantifying the missing sink for global organic carbon burial during a Cretaceous oceanic anoxic event, Earth Planet. Sci. Lett., 499, 83–94, 2018.
Pan, G. X., Cao, J. H., He, S. Y., Teng, Y. Z., and Xu, S. Y.: Sink effect of karst soil system on atmospheric CO2: evidence from field observation and simulation experiment, Earth Science Frontiers, 7, 580–587, 2000 (in Chinese).
Potter, C. S. and Klooster, S. A.: North American carbon sink, Science, 283, 1815, 1999.
Rustad, L. E., Huntington, T. G., and Boone, R. D.: Controls on soil respiration: implications for climate change, Biogeochemistry, 49, 1–6, 2000.
Solomon, D. K. and Cerling, T. E.: The annual carbon dioxide cycle in a montane soil: observation, modeling and implications for weathering, Water Resour. Res., 23, 2257–2265, 1987.
Sundquist, E. T.: The global carbon budget, Science, 259, 934–941, 1993.
Teir, S., Eloneva, S., Fogelholm, C. J., and Zevenhoven, R.: Stability of calcium carbonate and magnesium carbonate in rainwater and nitric acid solutions, Energ. Convers. Manage., 47, 3059–3068, 2006.
Warren, W. W. and Michael, J. P.: Origin and distribution of carbon dioxide in the unsaturated zone of the southern high plains of texas, Water Resour. Res., 20, 647–659, 1984.
Xu, S. Y. and He, S. Y.: The CO2 regime of soil profile and its drive to dissolution of carbonate rock, Carsologica Sinica, 18, 50–57, 1996 (in Chinese).
Yoyam, R. and Dani, O.: Stochastic modeling of unsaturated flow in heterogeneous soil with water uptake by plant roots: the parallel columns model, Water Resour. Res., 29, 619–631, 1993.
Zeng, S. W. and Zheng, L. P.: Mathematical model of soil CO2 transference in karst area, Shanghai Environmental Sciences, 21, 712–715, 2002 (in Chinese).
missing sink.